B cells present a double-sided effect in digestive system tumors: a review for tumor microenvironment
Introduction
Recently, the immune mechanism and changes of tumors is deeply exploring. The function of T lymphocytes (T cells) has been studied in depth and applied in clinical practice, such as immune checkpoint inhibitors. Although some immunotherapy strategies targeting B lymphocytes (B cells) have shown therapeutic effects in some studies, there are still many problems and deficiencies to be solved. For example, the identification of regulatory sites of B cell immune function, the prevention of up-regulation of cancer-promoting functions such as immune escape, the way of combining with other treatments to improve the therapeutic effect, etc. Therefore, it is of great significance to further study the mechanism of B cells in cancer and explore new immunotherapy strategies to improve the therapeutic effect of cancer.
In this review, the differentiation and types of B cells, the classification and function of antibodies, and some immune structures were described first. Next, according to the anatomy, B cells’ roles and functions in esophageal, liver, colorectal, gastric and pancreatic cancer were shown. Through the B cells’ dual function in tumors and the function changes in B cell associated with different organs, some relevant influencing factors were inferred. This review is expected to promote the understanding of the mechanism of B cells in cancer and help to realize the practice of B cells in immunotherapy.
The differentiation process of B cells
B cells, which primarily develop in the bone marrow, are humoral immunity cells that secrete antibodies for immune effects. Specifically, B cells arise from progenitor B cells (Pro-B cells) located in the fetal liver hematopoietic cells’ island before migrating to the bone marrow (1). Upon the initiation of heavy chain expression by Pro-B cells, precursor B cells (Pre-B cells) differentiate and lack the capacity to react to antigens or perform immune functions (2). At this stage, differentiation antigens specific to B cells, such as major histocompatibility complex (MHC), CD19, and CD20, manifest (3). Following light chain gene rearrangement, immature B cells (Bµ cells) differentiate from Pre-B, possessing intact IgM molecules capable of binding to antigens. When Bµ cells are bound to antigens, they undergo inhibition, which prevents their maturation. This mechanism is recognized as a contributing factor to the formation of B cell self-tolerance (4). After undergoing negative selection in the bone marrow, immature B cells migrate into the circulatory system and undergo maturation into transitional B cells within the spleen (5). In the initial stages, transitional B cells are designated as T1 B cells and could be found in the spleen, bone marrow, and the peripheral arterial lymphatic sleeves (PALS) outside of hair follicles (6). Through signal stimulation mediated by the B-cell activating factor (BAFF) family, T1 B cells differentiate into T2 B cells. Following their maturation, T2 B cells undergo differentiation into two distinct subtypes, namely naive B cells (NBC) and marginal zone B cells (MZB) (7). Following stimulation, NBC undergoes further differentiation into follicular B cells (FOB) as well as germinal center B cells (GCB) (Figure 1).
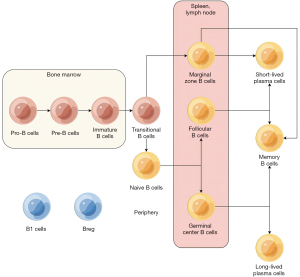
The final stage prior to the commencement of immune function by B cells is activation. Based on the reliance of their activation on T cells, B cells can be classified into two categories (8). T-cell-independent cells, including MZB, FOB, B1 cells, and regulatory B cells (Breg), constitute one such group (9). B1 cells, which are classified as innate lymphoid cells, are capable of recognizing nonprotein antigens (10). B1 cells are present in low quantities, which is not addressed in this review. Rather, conventional B cells undergo T-cell-dependent activation and differentiate into plasma cells (PC) and memory B cells (MBC) (11). The activation of B cells is facilitated by mature T follicular helper cells (TFH), which provide CD40 ligands and interleukin-21 (IL-21). Upon activation, B cells undergo proliferation and differentiation, with a significant proportion differentiating into PCs (12). This differentiation is marked by the appearance of surface markers such as plasma cell antigen-1 (PCA-1), which replace the original B cell surface markers (13). During class conversion, the immunoglobulin (Ig) produced by B cells transformed from IgM into IgG, IgA, or IgE. Meanwhile, a small subset of activated B cells undergoes differentiation into MBC, which exhibits limited proliferation and differentiation capabilities and can persist for several months to years (14). Upon encountering the same antigen, MBC rapidly activates and differentiates, exhibiting a short incubation period, high antibody (Ab) levels, and prolonged maintenance.
Immunoglobulin
The glycoprotein known as antibody is generated by B cells upon stimulation by antigens and is present in bodily fluids (15). It serves as a crucial effector molecule that facilitates humoral immunity by selectively binding to corresponding antigens. The heavy chains of antibody are classified into five categories, namely µ, δ, γ, α, and ε chain, which correspond to the five types of antibodies, namely IgM, IgG, IgD, IgA, and IgE (16). As a result, the identification of various B cell subsets can be achieved by discerning the different types of antibodies they produce.
The human body produces IgM as the initial antibody to combat new infections, comprising approximately 10% of normal serum Ig content (17). IgM serves as a receptor on the B cell membrane, receiving antigen stimulation. While a single molecule of IgM exhibits higher bactericidal and conditioning effects than other antibodies, its protective efficacy is limited due to its low blood concentration, short half-life, rapid clearance, and weak tissue penetration (18).
IgG is the most prevalent antibody type present in all bodily fluids and contributes significantly to humoral immunity (19). IgG serves as the primary antibody in re-immune response and possesses various functions, including phagocytosis, neutralization of toxins and viruses, mediation of antibody-dependent cell-mediated cytotoxicity (ADCC), and activation of the classical pathway of complement (20). Additionally, IgG is the sole antibody capable of crossing the placenta, thereby safeguarding newborns during the initial months of life. IgG comprises four subgroups, with IgG4 exhibiting a close association with the digestive system.
The molecular structure of IgD is remarkably similar to that of IgG, and it functions as a surface antigen receptor on B cells (21). However, due to its low concentration in normal human serum, research on IgD remains limited.
IgA is present in mucous membranes, including the respiratory and digestive tracts, as well as in bodily fluids such as saliva, tears, and breast milk (22). As a monomer, IgA functions to eliminate antigens in a noninflammatory manner and maintain internal stability. Secretory IgA, synthesized locally in gut-associated lymphoid tissue (GALT), exists as a dimer and serves a crucial role in immune defense (23).
IgE is present in its monomeric form and exhibits the lowest serum concentration among the five classes of Ig (24). It is predominantly localized in the respiratory tract and intestinal mucosa. However, IgE is essential in allergic responses and provides protection against parasitic infestations.
Immune structure
Immune composition of the digestive system
The immune system, which encompasses immune cells, tissues, and organs, serves as a protective barrier to the human body (25). Within the digestive system, the spleen and intestine play crucial roles as components of the immune system. The spleen functions not only as an immune site and storage location for immune cells but also as a synthesizer of immune molecules, including complement (26). The intestinal immune response is comprised of inherent immunity, adaptive immunity, and mucosal immunity.
GALT plays a pivotal role in intestinal immunity, acting as the principal mediator. It encompasses a diverse array of components, including Peyer’s patches (PP), independent lymphoid follicles, mesenteric lymph nodes, and lymphocytes located in the mucosal lamina propria (27). PP, a collection of lymphatic follicles within the small intestine’s mucosa, is comprised of B and T cells and is enveloped by a stratum of microfold cells referred to as M cells (28). These M cells possess the ability to recognize antigens within the gastrointestinal tract, phagocytose viruses and pathogens, and present ingested intestinal antigens to immune cells (29). Activated immune cells undergo homing to the lamina propria of the intestinal mucosa, where they differentiate into IgA-secreting PCs or effector T cells, thereby contributing to the local immune response of the intestinal tract.
Tertiary lymphoid structure (TLS)
TLS, which are anomalous lymphoid organs that emerge in non-lymphoid tissues, are commonly detected in a diverse range of persistent inflammatory ailments such as inflammatory diseases, autoimmune and infectious diseases, transplanted organs, and tumors (30). TLS is comprised of B cells, enveloped by T cells, and additionally encompasses TFH, dendritic cells, fibroblasts, blood vessels, and other cellular and structural components (31). TLS has been detected in the matrix, invasive margin, and core of various tumor types, particularly in proximity to tumors. Researches have demonstrated that TLS plays a pivotal role in the immune microenvironment by conferring distinct T cell phenotypes and that T cells exhibit dysfunctional molecular phenotypes in tumors lacking TLS (32).
Currently, TLS is widely regarded as a reliable indicator of tumor therapeutic immune response in numerous studies, thereby facilitating the promotion of antitumor immune response and positively impacting the overall survival (OS) and disease-free survival (DFS) of patients (33). Additionally, TLS can exert an influence on tumor invasion and metastasis.
B cells in cancers
Consistent with most studies, high B cell infiltration is positively correlated with tumor prognosis. However, some studies have shown that high B cell infiltration is unfavorable, especially in digestive system tumors. Therefore, the following takes digestive system cancers as the main direction, and discuss in detail the function and role of B cells in various cancers (Figure 2, Table 1).
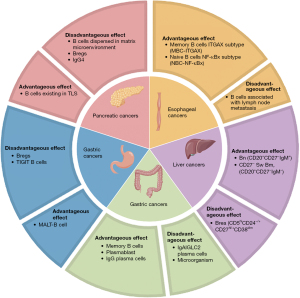
Table 1
Tumors | B cells | Functions | Ref. |
---|---|---|---|
Esophageal cancers | NBC-NF-κB | Enhance T cell function | (34) |
MBC-ITGAX | Promote B cell maturation, activation, production of cytokines | (34) | |
GC1 B | Prompt bad prognosis | (35) | |
GC2 B | Prompt good prognosis | ||
Liver cancers | Bn | Contribute to T cell activation | (36) |
CD27 Sw Bm | Produces antibodies (IgG or IgA) to promote humoral immunity | ||
PC | Negative regulatory T cells | (37) | |
Breg | Effectively inhibits tumor-specific T cell immunity, and promote tumor growth through IL-10 | (38) | |
Increased production of IL-40 and TGF-β promotes tumors progression, thereby reducing TNF-α levels and reducing anti-tumor immune responses | (39) | ||
Colorectal cancers | MBC | Antigen presentation, chemokine secretion | (40) |
PC | Secrete a lot of IgG | ||
Transitional B cells | Suggest loss of immune tolerance | (41) | |
Plasmablast | Suppressing harmful Th17 inflammation | (42) | |
Inhibition of IFN-γ and TNF-α | (43) | ||
IgAIGLC2 PCs | Enhance allograft rejection, IFN-α reaction, IFN-γ reaction and inflammatory reaction of tumor-derived cells | (44) | |
Gastric cancers | TIGIT B cells | Shape immune invasive structures to promote tumor progression | (45) |
MALT-B cells | Activation of complement pathway promotes antitumor immunity | (46) | |
Breg | Inhibit the proliferation of autologous CD4 T cells, inhibit the production of IFN-γ by CD4 T cells, and enhance immune escape | (47) | |
Pancreatic cancers | IgG1 MBC | Complement activation, binding of Fc receptors on the surface of effector cells and involvement of ADCC | (48) |
Breg | Secretion of IL-35 promotes immunosuppression and tumor growth | (49) | |
Expresses high levels of PD-L1, resulting in reduced T cells proliferation and IFN-γ secretion | (50) | ||
IgG4 PCs | Positively correlated with poor histological grade and overall survival | (51) |
NBC, naive B cell; NF-κB, nuclear factor κB; IL-10, interleukin 10; TNF-α, tumor necrosis factor α; MBC, memory B cell; GCB, germinal center B cell; PC, plasma cell; MALT, mucosa-associated lymphoid tissue; TIGIT, T-cell immune receptor with immunoglobulin and ITIM domain; ADCC, antibody-dependent cell-mediated cytotoxicity; PD-L1, programmed cell death ligand 1; IFN-γ, interferon γ; TGF-β, transforming growth factor β.
Esophageal cancers
Esophageal cancer represents a prevalent and lethal form of cancer, histologically classified into two subtypes: esophageal adenocarcinoma (EAC) and esophageal squamous cell carcinoma (ESCC) (52).
ESCC, which constitutes approximately 90% of global esophageal cancer cases, has been studied through single-cell sequencing of tumor samples to obtain its transcription map. The tumors exhibit a presence of common immune cells, such as T cells, natural killer (NK) cells, monocytes/macrophages, dendritic cells (DC), B cells, and mast cells. Mature TLS, which is rich in various immune cells, significantly enhances the anti-tumor immunity of ESCC (53). However, ESCC displays a higher infiltration of T cells and monocytes/macrophages and a lower presence of B cells compared to normal tissues and other cancers (54). Despite the low quantity of B cells, they exhibit a diverse range. B cells in ESCC exhibit a diverse range of subgroups, totaling at least 20, which can be further classified into ten distinct subtypes based on their respective functions. These subtypes include NBC, GC1, GC2, PC, MBC, interferon (IFN)-induced, Innate, double negative (DN), mature, and activated B cells (35). Furthermore, data revealed the identification of novel and unique B cell subtypes, such as NBC-NF-κB, MBC-ITGAX, and other four plasmablast subtypes (34). Notably, the gene-phenotype of NBC-NF-κBx has been shown to have an enhanced effect on T cells. The MBC-ITGAX subtype has demonstrated significant antitumor benefits.
The MBC-ITGAX subtype is characterized by an enrichment of tumor necrosis factor-α (TNF-α) signaling through the nuclear factor κB (NF-κB) pathway and the IL-2-STAT5 signaling pathway (55). The NF-κB pathway plays a role in B cell maturation and adaptive immunity, positively regulating B cell activation and cytokine production through TNF-α signal transduction (56). The presence of NF-κB related genes is indicative of a favorable prognosis in esophageal cancer.
When considered in conjunction with other clinical studies, B cell infiltration is positively associated with a favorable prognosis (57). While some B cells that are associated with lymph node metastasis and poor prognosis have been identified, their presence is insufficient to diminish the tumor-killing effect of high B cell infiltration (35).
Liver cancers
Numerous risk factors have been identified as contributing to the development of liver fibrosis or sclerosis, which is often accompanied by substantial immune infiltration and ultimately culminates in the emergence of liver cancer (58).
Throughout the progression of hepatocellular carcinoma (HCC), the concentration of B cells in liver tissue varies, with B cells being abundant in normal liver tissue. Upon the transformation of tissue to cirrhosis, the proportion of immune cells, namely T cells (1.40%), B cells (5.49%), and macrophages (0.41%), decreases (37). Then the proportion of B cells in HCC increases by 18.85%. B cells predominantly infiltrate the tumor margin and are comprised of five subgroups: NBCs (Bn, CD20+CD27−IgM+), IgM+ MBCs (IgM+ Bm, CD20+CD27+IgM+), CD27− isotype-switched MBCs (CD27− Sw Bm, CD20+CD27−IgM−) and CD27+ isotype-switched MBCs (CD27+ Sw Bm, CD20+CD27+IgM−) and PCs (CD20−CD24−CD27hiCD38hi) (36). Researches have established that a high density of B cell infiltration is positively correlated with small tumor size, absence of vascular invasion, and increased density of CD8+ T cells (59). Bn and CD27− Sw Bm, in particular, showed better survival correlation. Simultaneously, a substantial correlation exists between elevated B cell infiltration and prolonged survival time, with patients exhibiting high B cell density presenting with better clinicopathological features, providing compelling evidence for the antitumor effect of B cells (60).
Nevertheless, reports acknowledging the acceleration of tumor progression by B cells cannot be disregarded, with Breg playing a pivotal role in this regard (61). In advanced HCC, the percentage of Breg in tumor tissues significantly increased, constituting approximately 10% of all B cells. These Breg exhibited a distinctive phenotype: CD5hiCD24−/+CD27hi/+CD38dim (38). These Breg have been shown to effectively inhibit tumor-specific T cell immunity and promote tumor growth in vivo through IL-10 (62). Furthermore, PC is recognized to exhibit an adverse regulatory association with T cells, while the quantity of B cells and FOXP3 Treg cells has been verified to display a positive correlation (39).
Colorectal cancers
The prevalence and fatality rate of colorectal cancer (CRC) persist in an upward trajectory. Owing to its inconsequential clinical manifestations and absence of specificity, over 80% of patients are diagnosed in the intermediate and advanced stages, with over 40% of patients experiencing liver and lung metastases (63).
In CRC, macrophages constitute the predominant immune cell type, followed by T cells, B cells, bone marrow cells, and mast cells. In comparison to normal tissues, tumors exhibit enrichment of T cells, while B cells are depleted (44). The identification of B cells in tumor tissues reveals their classification into NBC, MBC, GCB cells, IgA PCs, and IgG PCs (40). Notably, the proportion of effector MBC and plasmablasts is significantly elevated in CRC. MBC exhibits comparable functions to NBC and possesses abundant antigen presentation and chemokine signaling pathways (64). The expression of genes related to antigen presentation is consistently elevated. Tumor tissues exhibit a higher abundance of IgG PCs, with infiltration levels decreasing from the tumor center to the periphery (65). Additionally, the IgG/IgA PC ratio increases.
The elevated density of infiltrating B cells is associated with the lower stage tumor and the right colon, which is commonly believed to exhibit a more favorable prognosis and lower malignancy degree in tumors (66). Furthermore, patients with heightened B cell infiltration demonstrate a noteworthy increase in survival duration. In CRC, B cells and T cells exhibit a strong positive correlation due to the cooperative nature of immune cells, which is advantageous for tumor eradication (40).
Nevertheless, the role of B cells in tumor elimination becomes intricate due to the abundance of microorganisms and IgA in the intestine (41). The potential etiology of these outcomes may be attributed to the destruction of tumor tissue to the intestinal barrier. The disruption of barrier function may impede the negative selection of autoantigen-specific B cells, leading to the accumulation of immature B cells in tissues, including elevated levels of T1 and T2 B cells (67). These immature B cells are characterized by the expression of IgM and exhibit inadequate immune function when compared to IgG. Furthermore, the impairment of the barrier may result in the loss of intestinal tolerance to microbial antigens (68).
IgA PCs are localized specifically in the mucosa of CRC, and the presence of IgA GLC2 PCs has been linked to a negative prognosis (44). These cells have been found to augment allograft rejection, interferon-α (IFN-α) and IFN-γ reactions, and inflammatory responses, suggesting their involvement in promoting tumor-associated inflammation (43).
Gastric cancers (GC)
GC is a malignancy that arises from the epithelium of the gastric mucosa and is the most common digestive tract malignancy. The immune response plays a significant role in the initiation and progression of GC. The majority of B cells infiltrating tumors in GC are present in the form of TLS surrounding the tumor, as well as in the normal gastric mucosa surrounding the tumor (69).
Upon identification of GC tissues, B cells are classified into various subtypes, including PCs, B cells originating from mucosa-associated lymphoid tissue (MALT), NBC, FOB cells, MBC, inflammatory B cells, and tumor-associated B cells (46). In GC with a high abundance of TLS, the proportion of NBC is low, while GCB cells and PCs are increased. As the predominant subtype, GCB cells exhibit strong activation (69). Furthermore, a significant quantity of MALT-B cells exhibiting specificity were identified within TLS. These MALT-B cells are almost only found in GC tissues that contain TLS, which belong to a terminally differentiated subset of IgA PCs (70). The functions of MALT-B cells may be associated with complement activation, immune response, and B cell activation. Additionally, researches have demonstrated that the infiltration of B cells within GC tissue is positively correlated with the infiltration of T cells, but negatively correlated with TH1 cells, TH2 cells, and Treg cells (71). The elevated degree of B cell infiltration is associated with extended OS and DFS, with particular emphasis on the heightened infiltration of MBC and PCs, signifying a more favorable prognosis (72).
However, some studies indicate that B cells may act in parallel to promote tumorigenesis. Breg in GC tissue was significantly higher than that in normal tissue. Breg in the peripheral blood of GC patients was significantly higher than that of the healthy control group, and Breg decreased significantly after an operation (47). Breg was found to be enriched in CD19CD24CD27 B cell subsets in GC patients. The CD19+CD24hiCD27+ B cells have been shown to possess the ability to inhibit the proliferation of CD4 T cells and suppress the secretion of IFN-γ by autologous T cells (47,73). Breg has been observed to transform CD4+CD25− effector T cells into CD4+FoxP3+ Tregs through TGF-β1. Additionally, the T-cell immune receptor with immunoglobulin and ITIM domain (TIGIT), which is highly expressed, has been found to be associated with promoting tumor progression by shaping an immune-invasive structure (74). TIGIT-B cells have been observed to infiltrate into and around the tumor in GC, and this has been suggested to be indicative of poor prognosis in GC (45).
Pancreatic cancers
Pancreatic cancer is a highly invasive malignant tumor with a 5-year survival rate of only 5–7%. Current treatment methods have proven little effective in treating this disease, likely due to the ability of pancreatic cancer cells to evade immune surveillance, develop drug resistance, and metastasize early (75).
In pancreatic cancer, the function of B cells remains a topic of controversy, with their impact on tumor growth dependent on their localization within tumor tissue. B cells present in TLS have demonstrated a tumor-killing effect, resulting in longer OS and DFS (76). Samples exhibiting high levels of TLS demonstrated upregulation of genes associated with the germinal center reaction, B cell activation, and proliferation. The germinal center reaction is enriched in IgG1 MBC, effector memory CD4+ T cells, and NK cells, indicating the presence of long-lasting immune memory (48).
Conversely, B cells dispersed within the matrix microenvironment exhibited an increase in the expression of immunosuppressive genes. In comparison to healthy individuals, patients with pancreatic cancer exhibited an expansion of Breg, which was associated with tumor stage and low survival rates (77). The expansion of Breg in pancreatic cancer patients is induced by the secretion of IL-18 by pancreatic cancer cells (78). In pancreatic cancers, Breg facilitates the conversion and recombination of pro-inflammatory IgG1 to anti-inflammatory IgG4, which is associated with a significant infiltration of IgG4 PCs and a negative correlation with histological grade and OS rate (79). The production of IgG4 is typically induced by a Th2 immune response driven by IL-10 and IL-4. Thus, the Breg-mediated conversion of IgG classes may serve as an alternative immune evasion mechanism.
Furthermore, in stark contrast to the invasive nature of pancreatic cancer, the majority of tumor volumes consist of cancer-associated fibroblasts (CAFs), extracellular matrix (ECM), and immune cells, collectively referred to as the “tumor matrix” (80). The presence of circulating plasmablasts can stimulate CAFs to produce a significant amount of collagen, thereby promoting tumor progression through increased matrix deposition (81).
Conclusions
In the tumor microenvironment, the function of T cells has been extensively investigated and effectively implemented in clinical practice. However, the functional investigation and clinical application of B cells, which serve as the primary mediator of humoral immunity, remain insufficient, because of the complex regulatory role of B cells. For tumors, B cells’ bidirectional regulation is notably pronounced. Undoubtedly, B cells exhibit tumoricidal activity in the majority of tumors. However, in select tumors, particularly those of the digestive system, a high degree of B cell infiltration is associated with a poorer prognosis (82). Notably, in tumors of the esophagus and liver, a high degree of B cell infiltration is generally indicative of a favorable prognosis. In colorectal and gastric tumors, the prognostic implications of high B cell infiltration are equivocal. Finally, in pancreatic cancer, B cells are frequently linked with unfavorable outcomes.
In conjunction with additional research, it is our assertion that the elements contributing to the heightened tumor-promoting properties of B cells in digestive neoplasms encompass IgG4, microorganisms, IgA, and Breg. Although the function of IgG4 immunoglobulin is still being investigated, its association with autoimmune diseases is well-established. IgG4-related diseases are characterized by attacking various tissues and organs, with a predilection for glandular and digestive system involvement, particularly the pancreas (83). This phenomenon may elucidate the atypical elevation of IgG4 and the detrimental consequences of heightened B cell infiltration in pancreatic cancer. Microorganisms are a distinct presence within the digestive tract, whose carcinogenic impact has been established, with particular emphasis on the induction of GC by Helicobacter pylori (HP). As previously discussed in the context of CRC, the impairment of the intestinal barrier can have a complex effect on immune function through the alteration of B cell function by beneficial intestinal microorganisms. In contrast to other tissues, the intestinal mucosa is abundant in IgA and exerts a significant influence on the immune milieu of the intestine. As previously stated, certain subsets of IgA PCs have been definitively linked to poorer tumor prognoses. Additionally, IgA PCs in pancreatic cancer secrete a greater quantity of cytokines (e.g., IL-10) that facilitate the development of Breg (84). The functionality and regulation of Breg have been extensively investigated. Breg’s role in promoting tumor growth in B cells is a direct responsibility. The remaining three factors, either as inducers of Breg or as parallel influencers, contribute to tumor development. This phenomenon partially accounts for the prevalence of Breg in digestive system tumors. Consequently, immunotherapy for B cells should prioritize the inhibition of Breg.
Acknowledgments
Funding: This present work was funded by
Footnote
Peer Review File: Available at https://tgh.amegroups.com/article/view/10.21037/tgh-23-127/prf
Conflicts of Interest: All authors have completed the ICMJE uniform disclosure form (available at https://tgh.amegroups.com/article/view/10.21037/tgh-23-127/coif). X.L. reports the funding from the National Natural Science Foundation of China (No. 81972671). The other authors have no conflicts of interest to declare.
Ethical Statement:
Open Access Statement: This is an Open Access article distributed in accordance with the Creative Commons Attribution-NonCommercial-NoDerivs 4.0 International License (CC BY-NC-ND 4.0), which permits the non-commercial replication and distribution of the article with the strict proviso that no changes or edits are made and the original work is properly cited (including links to both the formal publication through the relevant DOI and the license). See: https://creativecommons.org/licenses/by-nc-nd/4.0/.
References
- Jain N. The early life education of the immune system: Moms, microbes and (missed) opportunities. Gut Microbes 2020;12:1824564. [Crossref] [PubMed]
- Küppers R, Stevenson FK. Critical influences on the pathogenesis of follicular lymphoma. Blood 2018;131:2297-306. [Crossref] [PubMed]
- Deak D, Pop C, Zimta AA, et al. Let's Talk About BiTEs and Other Drugs in the Real-Life Setting for B-Cell Acute Lymphoblastic Leukemia. Front Immunol 2019;10:2856. [Crossref] [PubMed]
- Gonzalez-Martin A, Adams BD, Lai M, et al. The microRNA miR-148a functions as a critical regulator of B cell tolerance and autoimmunity. Nat Immunol 2016;17:433-40. [Crossref] [PubMed]
- Härzschel A, Zucchetto A, Gattei V, et al. VLA-4 Expression and Activation in B Cell Malignancies: Functional and Clinical Aspects. Int J Mol Sci 2020;21:2206. [Crossref] [PubMed]
- Cieniewicz B, Santana AL, Minkah N, et al. Interplay of Murine Gammaherpesvirus 68 with NF-kappaB Signaling of the Host. Front Microbiol 2016;7:1202. [Crossref] [PubMed]
- Chen Y, Yu M, Podd A, et al. A critical role of Rap1b in B-cell trafficking and marginal zone B-cell development. Blood 2008;111:4627-36. [Crossref] [PubMed]
- Groom J, Mackay F. B cells flying solo. Immunol Cell Biol 2008;86:40-6. [Crossref] [PubMed]
- Demaria MC, Yeung L, Peeters R, et al. Tetraspanin CD53 Promotes Lymphocyte Recirculation by Stabilizing L-Selectin Surface Expression. iScience 2020;23:101104. [Crossref] [PubMed]
- Largeot A, Pagano G, Gonder S, et al. The B-side of Cancer Immunity: The Underrated Tune. Cells 2019;8:449. [Crossref] [PubMed]
- Thomas MJ, Klein U, Lygeros J, et al. A Probabilistic Model of the Germinal Center Reaction. Front Immunol 2019;10:689. [Crossref] [PubMed]
- Zielinski CE, Corti D, Mele F, et al. Dissecting the human immunologic memory for pathogens. Immunol Rev 2011;240:40-51. [Crossref] [PubMed]
- Musto P, D'Auria F. The clinical and biological role of CD20 membrane antigen modulation under immunotherapy with anti-CD20 monoclonal antibody rituximab in lymphoprolipherative neoplastic disorders. Expert Opin Biol Ther 2011;11:551-7. [Crossref] [PubMed]
- Garman L, Smith K, Farris AD, et al. Protective antigen-specific memory B cells persist years after anthrax vaccination and correlate with humoral immunity. Toxins (Basel) 2014;6:2424-31. [Crossref] [PubMed]
- Parray HA, Shukla S, Samal S, et al. Hybridoma technology a versatile method for isolation of monoclonal antibodies, its applicability across species, limitations, advancement and future perspectives. Int Immunopharmacol 2020;85:106639. [Crossref] [PubMed]
- Wang C, Lu Y, He K, et al. Comparative proteomics analyses of whey proteins from breastmilk collected from two ethnic groups in northeast China. Food Chem X 2023;17:100568. [Crossref] [PubMed]
- Choi J, Gani AW, Bechstein DJB, et al. Portable, one-step, and rapid GMR biosensor platform with smartphone interface. Biosens Bioelectron 2016;85:1-7. [Crossref] [PubMed]
- Cai P, Weerakoon KG, Mu Y, et al. Comparison of Kato Katz, antibody-based ELISA and droplet digital PCR diagnosis of schistosomiasis japonica: Lessons learnt from a setting of low infection intensity. PLoS Negl Trop Dis 2019;13:e0007228. [Crossref] [PubMed]
- Narayanaperumal J, D'souza A, Miriyala A, et al. A randomized double blinded placebo controlled clinical trial for the evaluation of green coffee extract on immune health in healthy adults. J Tradit Complement Med 2022;12:455-65. [Crossref] [PubMed]
- Chahar MK, Sanjaya Kumar DS, Lokesh T, et al. In-vivo antioxidant and immunomodulatory activity of mesuol isolated from Mesua ferrea L. seed oil. Int Immunopharmacol 2012;13:386-91. [Crossref] [PubMed]
- Rousseaux A, Brosseau C, Bodinier M. Immunomodulation of B Lymphocytes by Prebiotics, Probiotics and Synbiotics: Application in Pathologies. Nutrients 2023;15:269. [Crossref] [PubMed]
- Niedźwiedź A, Pius-Sadowska E, Kawa M, et al. IgA-Based Secretory Response in Tears of COVID-19 Patients: A Potential Biomarker of Pro-Inflammatory State in Course of SARS-CoV-2 Infection. Pathogens 2022;11:1098. [Crossref] [PubMed]
- Meresse B, Malamut G, Cerf-Bensussan N. Celiac disease: an immunological jigsaw. Immunity 2012;36:907-19. [Crossref] [PubMed]
- Karnowski A, Achatz-Straussberger G, Klockenbusch C, et al. Inefficient processing of mRNA for the membrane form of IgE is a genetic mechanism to limit recruitment of IgE-secreting cells. Eur J Immunol 2006;36:1917-25. [Crossref] [PubMed]
- Pan X, Li C, Feng J. The role of LncRNAs in tumor immunotherapy. Cancer Cell Int 2023;23:30. [Crossref] [PubMed]
- Bao Z, Zhang Z, Zhou G, et al. Novel Mechanisms and Therapeutic Targets for Ischemic Stroke: A Focus on Gut Microbiota. Front Cell Neurosci 2022;16:871720. [Crossref] [PubMed]
- Kaneko M, Akiyama Y, Takimoto H, et al. Mechanism of up-regulation of immunoglobulin A production in the intestine of mice unresponsive to lipopolysaccharide. Immunology 2005;116:64-70. [Crossref] [PubMed]
- Kimura S, Nakamura Y, Kobayashi N, et al. Osteoprotegerin-dependent M cell self-regulation balances gut infection and immunity. Nat Commun 2020;11:234. [Crossref] [PubMed]
- Pfeiffer JK. Innate host barriers to viral trafficking and population diversity: lessons learned from poliovirus. Adv Virus Res 2010;77:85-118. [Crossref] [PubMed]
- Greenberg J, Limberg J, Verma A, et al. Metastatic pancreatic neuroendocrine tumors feature elevated T cell infiltration. JCI Insight 2022;7:e160130. [Crossref] [PubMed]
- Qin M, Jin Y, Pan LY. Tertiary lymphoid structure and B-cell-related pathways: A potential target in tumor immunotherapy. Oncol Lett 2021;22:836. [Crossref] [PubMed]
- Cabrita R, Lauss M, Sanna A, et al. Tertiary lymphoid structures improve immunotherapy and survival in melanoma. Nature 2020;577:561-5. [Crossref] [PubMed]
- Munoz-Erazo L, Rhodes JL, Marion VC, et al. Tertiary lymphoid structures in cancer - considerations for patient prognosis. Cell Mol Immunol 2020;17:570-5. [Crossref] [PubMed]
- Nakamura S, Ohuchida K, Ohtsubo Y, et al. Single-cell transcriptome analysis reveals functional changes in tumour-infiltrating B lymphocytes after chemotherapy in oesophageal squamous cell carcinoma. Clin Transl Med 2023;13:e1181. [Crossref] [PubMed]
- Jia Y, Zhang B, Zhang C, et al. Single-Cell Transcriptomic Analysis of Primary and Metastatic Tumor Ecosystems in Esophageal Squamous Cell Carcinoma. Adv Sci (Weinh) 2023;10:e2204565. [Crossref] [PubMed]
- Zhang Z, Ma L, Goswami S, et al. Landscape of infiltrating B cells and their clinical significance in human hepatocellular carcinoma. Oncoimmunology 2019;8:e1571388. [Crossref] [PubMed]
- Zhang S, Liu Z, Wu D, et al. Single-Cell RNA-Seq Analysis Reveals Microenvironmental Infiltration of Plasma Cells and Hepatocytic Prognostic Markers in HCC With Cirrhosis. Front Oncol 2020;10:596318. [Crossref] [PubMed]
- Xiao X, Lao XM, Chen MM, et al. PD-1hi Identifies a Novel Regulatory B-cell Population in Human Hepatoma That Promotes Disease Progression. Cancer Discov 2016;6:546-59. [Crossref] [PubMed]
- Feng Y, Liu L, Li J, et al. Systematic characterization of the tumor microenvironment in Chinese patients with hepatocellular carcinoma highlights intratumoral B cells as a potential immunotherapy target. Oncol Rep 2022;47:38. [Crossref] [PubMed]
- Xia J, Xie Z, Niu G, et al. Single-cell landscape and clinical outcomes of infiltrating B cells in colorectal cancer. Immunology 2023;168:135-51. [Crossref] [PubMed]
- Noble A, Pring ET, Durant L, et al. Altered immunity to microbiota, B cell activation and depleted γδ/resident memory T cells in colorectal cancer. Cancer Immunol Immunother 2022;71:2619-29. [Crossref] [PubMed]
- Mao H, Pan F, Wu Z, et al. CD19loCD27hi Plasmablasts Suppress Harmful Th17 Inflammation Through Interleukin 10 Pathway in Colorectal Cancer. DNA Cell Biol 2017;36:870-7. [Crossref] [PubMed]
- Mao H, Pan F, Wu Z, et al. Colorectal tumors are enriched with regulatory plasmablasts with capacity in suppressing T cell inflammation. Int Immunopharmacol 2017;49:95-101. [Crossref] [PubMed]
- Wang W, Zhong Y, Zhuang Z, et al. Multiregion single-cell sequencing reveals the transcriptional landscape of the immune microenvironment of colorectal cancer. Clin Transl Med 2021;11:e253. [Crossref] [PubMed]
- Liu H, Wu J, Xu X, et al. Peritumoral TIGIT(+)CD20(+) B cell infiltration indicates poor prognosis but favorable adjuvant chemotherapeutic response in gastric cancer. Int Immunopharmacol 2022;108:108735. [Crossref] [PubMed]
- Jia L, Wang T, Zhao Y, et al. Single-cell profiling of infiltrating B cells and tertiary lymphoid structures in the TME of gastric adenocarcinomas. Oncoimmunology 2021;10:1969767. [Crossref] [PubMed]
- Murakami Y, Saito H, Shimizu S, et al. Increased regulatory B cells are involved in immune evasion in patients with gastric cancer. Sci Rep 2019;9:13083. [Crossref] [PubMed]
- J Gunderson A. Germinal center reactions in tertiary lymphoid structures associate with neoantigen burden, humoral immunity and long-term survivorship in pancreatic cancer. Oncoimmunology 2021;10:1900635. [Crossref] [PubMed]
- Mirlekar B, Michaud D, Lee SJ, et al. B cell-Derived IL35 Drives STAT3-Dependent CD8(+) T-cell Exclusion in Pancreatic Cancer. Cancer Immunol Res 2020;8:292-308. [Crossref] [PubMed]
- Khan AR, Hams E, Floudas A, et al. PD-L1hi B cells are critical regulators of humoral immunity. Nat Commun 2015;6:5997. [Crossref] [PubMed]
- Liu Q, Niu Z, Li Y, et al. Immunoglobulin G4 (IgG4)-positive plasma cell infiltration is associated with the clinicopathologic traits and prognosis of pancreatic cancer after curative resection. Cancer Immunol Immunother 2016;65:931-40. [Crossref] [PubMed]
- Zhang W, Luo J, Xiao Z, et al. USP36 facilitates esophageal squamous carcinoma progression via stabilizing YAP. Cell Death Dis 2022;13:1021. [Crossref] [PubMed]
- Nakamura S, Ohuchida K, Hayashi M, et al. Tertiary lymphoid structures correlate with enhancement of antitumor immunity in esophageal squamous cell carcinoma. Br J Cancer 2023;129:1314-26. [Crossref] [PubMed]
- Zheng Y, Chen Z, Han Y, et al. Immune suppressive landscape in the human esophageal squamous cell carcinoma microenvironment. Nat Commun 2020;11:6268. [Crossref] [PubMed]
- Hipp N, Symington H, Pastoret C, et al. IL-2 imprints human naive B cell fate towards plasma cell through ERK/ELK1-mediated BACH2 repression. Nat Commun 2017;8:1443. [Crossref] [PubMed]
- Kaileh M, Sen R. NF-κB function in B lymphocytes. Immunol Rev 2012;246:254-71. [Crossref] [PubMed]
- Chen Z, Zhao M, Liang J, et al. Dissecting the single-cell transcriptome network underlying esophagus non-malignant tissues and esophageal squamous cell carcinoma. EBioMedicine 2021;69:103459. [Crossref] [PubMed]
- He M, Peng A, Huang XZ, et al. Peritumoral stromal neutrophils are essential for c-Met-elicited metastasis in human hepatocellular carcinoma. Oncoimmunology 2016;5:e1219828. [Crossref] [PubMed]
- Shi JY, Gao Q, Wang ZC, et al. Margin-infiltrating CD20(+) B cells display an atypical memory phenotype and correlate with favorable prognosis in hepatocellular carcinoma. Clin Cancer Res 2013;19:5994-6005. [Crossref] [PubMed]
- Nie Y, Fan H, Li J, et al. Tertiary lymphoid structures: Associated multiple immune cells and analysis their formation in hepatocellular carcinoma. FASEB J 2022;36:e22586. [Crossref] [PubMed]
- Ye L, Zhang Q, Cheng Y, et al. Tumor-derived exosomal HMGB1 fosters hepatocellular carcinoma immune evasion by promoting TIM-1(+) regulatory B cell expansion. J Immunother Cancer 2018;6:145. [Crossref] [PubMed]
- Blair PA, Noreña LY, Flores-Borja F, et al. CD19(+)CD24(hi)CD38(hi) B cells exhibit regulatory capacity in healthy individuals but are functionally impaired in systemic Lupus Erythematosus patients. Immunity 2010;32:129-40. [Crossref] [PubMed]
- Ferreira D, Silva AP, Nobrega FL, et al. Rational Identification of a Colorectal Cancer Targeting Peptide through Phage Display. Sci Rep 2019;9:3958. [Crossref] [PubMed]
- Shlomchik MJ, Weisel F. Germinal center selection and the development of memory B and plasma cells. Immunol Rev 2012;247:52-63. [Crossref] [PubMed]
- Desbois M, Udyavar AR, Ryner L, et al. Integrated digital pathology and transcriptome analysis identifies molecular mediators of T-cell exclusion in ovarian cancer. Nat Commun 2020;11:5583. [Crossref] [PubMed]
- Edin S, Kaprio T, Hagström J, et al. The Prognostic Importance of CD20(+) B lymphocytes in Colorectal Cancer and the Relation to Other Immune Cell subsets. Sci Rep 2019;9:19997. [Crossref] [PubMed]
- Chen J, Pitmon E, Wang K. Microbiome, inflammation and colorectal cancer. Semin Immunol 2017;32:43-53. [Crossref] [PubMed]
- Bijelić B, Matić IZ, Besu I, et al. Celiac disease-specific and inflammatory bowel disease-related antibodies in patients with recurrent aphthous stomatitis. Immunobiology 2019;224:75-9. [Crossref] [PubMed]
- Yamakoshi Y, Tanaka H, Sakimura C, et al. Immunological potential of tertiary lymphoid structures surrounding the primary tumor in gastric cancer. Int J Oncol 2020;57:171-82. [Crossref] [PubMed]
- Lambrechts D, Wauters E, Boeckx B, et al. Phenotype molding of stromal cells in the lung tumor microenvironment. Nat Med 2018;24:1277-89. [Crossref] [PubMed]
- Ni Z, Xing D, Zhang T, et al. Tumor-infiltrating B cell is associated with the control of progression of gastric cancer. Immunol Res 2021;69:43-52. [Crossref] [PubMed]
- Yoshida T, Mei H, Dörner T, et al. Memory B and memory plasma cells. Immunol Rev 2010;237:117-39. [Crossref] [PubMed]
- Chen X, Cai C, Xu D, et al. Human Mesenchymal Stem Cell-Treated Regulatory CD23(+)CD43(+) B Cells Alleviate Intestinal Inflammation. Theranostics 2019;9:4633-47. [Crossref] [PubMed]
- Liudahl SM, Betts CB, Sivagnanam S, et al. Leukocyte Heterogeneity in Pancreatic Ductal Adenocarcinoma: Phenotypic and Spatial Features Associated with Clinical Outcome. Cancer Discov 2021;11:2014-31. [Crossref] [PubMed]
- Erkan M, Hausmann S, Michalski CW, et al. The role of stroma in pancreatic cancer: diagnostic and therapeutic implications. Nat Rev Gastroenterol Hepatol 2012;9:454-67. [Crossref] [PubMed]
- Castino GF, Cortese N, Capretti G, et al. Spatial distribution of B cells predicts prognosis in human pancreatic adenocarcinoma. Oncoimmunology 2016;5:e1085147. [Crossref] [PubMed]
- Minici C, Testoni S, Della-Torre E. B-Lymphocytes in the Pathophysiology of Pancreatic Adenocarcinoma. Front Immunol 2022;13:867902. [Crossref] [PubMed]
- Pylayeva-Gupta Y, Das S, Handler JS, et al. IL35-Producing B Cells Promote the Development of Pancreatic Neoplasia. Cancer Discov 2016;6:247-55. [Crossref] [PubMed]
- Candando KM, Lykken JM, Tedder TF. B10 cell regulation of health and disease. Immunol Rev 2014;259:259-72. [Crossref] [PubMed]
- Kadaba R, Birke H, Wang J, et al. Imbalance of desmoplastic stromal cell numbers drives aggressive cancer processes. J Pathol 2013;230:107-17. [Crossref] [PubMed]
- Falcomatà C, Bärthel S, Schneider G, et al. Context-Specific Determinants of the Immunosuppressive Tumor Microenvironment in Pancreatic Cancer. Cancer Discov 2023;13:278-97. [Crossref] [PubMed]
- Sim GC, Radvanyi L. The IL-2 cytokine family in cancer immunotherapy. Cytokine Growth Factor Rev 2014;25:377-90. [Crossref] [PubMed]
- Kempeneers D, Hauben E, De Haes P. IgG4-related skin lesions: case report and review of the literature. Clin Exp Dermatol 2014;39:479-83. [Crossref] [PubMed]
- Senturk ZN, Akdag I, Deniz B, et al. Pancreatic cancer: Emerging field of regulatory B-cell-targeted immunotherapies. Front Immunol 2023;14:1152551. [Crossref] [PubMed]
Cite this article as: Xu J, Lu W, Yang J, Liu X. B cells present a double-sided effect in digestive system tumors: a review for tumor microenvironment. Transl Gastroenterol Hepatol 2024;9:46.