Isosteviol plays a protective role on hepatic ischemia and reperfusion injury in mice through MAPK/NF-κB signaling pathway
Highlight box
Key findings
• Isosteviol (ISV) plays a protective role on hepatic ischemia and reperfusion (I/R) injury through mitogen-activated protein kinase (MAPK)/nuclear factor kappa-B (NF-κB) signaling pathway.
What is known and what is new?
• ISV displays potential therapeutic effects, including antioxidant, anti-inflammatory, anti-apoptotic, and neuroprotective activities.
• ISV exerts a protective effect against hepatic I/R injury.
What is the implication, and what should change now?
• The potential clinical therapeutic value and the specific molecular mechanism of ISV might be explored in future studies.
Introduction
Hepatic ischemia and reperfusion (I/R) injury is commonly encountered in various surgical procedures, such as cancer and liver transplantation, which is an important cause of liver dysfunction or failure after liver surgery (1,2). The characteristic of liver I/R is that tissue hypoxia caused by limited blood supply and reperfusion induces oxidative stress and immune response produces a large number of oxygen free radicals, leading to cellular apoptosis or necrosis (3,4). It has been studied that activation of Kupffer cells (KCs) can cause liver I/R injury and subsequently lead to the release of pro-inflammatory cytokines, including tumor necrosis factor (TNF)-α, interleukin (IL)-1β, and IL-6, these cytokines can damage the liver (5,6).
Apoptosis is a basic biological phenomenon of cells and a process of multi-gene regulation, and studies have implicated that apoptosis was involving in the pathogenesis of hepatic I/R injury (7,8). Reperfusion activates many proteins involved in apoptosis, such as protease caspase-3, BCL2-associated X protein (BAX) and downregulated anti-apoptotic protein B-cell lymphoma-2 (BCL-2). At present, this kind of injury can be reduced by reducing the release of oxygen free radicals and inflammatory cytokines (9).
Nuclear factor kappa-B (NF-κB) is a family of inducible transcription factors that can regulate a variety of biological processes, including immune and inflammatory responses cell growth and survival, and tumorigenesis (10,11). Mitogen-activated protein kinase (MAPK) pathway form a large family of serine/threonine kinases, which respond to a variety of extracellular and intracellular stimulation, and mediate a variety of biological processes, such as cell growth and differentiation, immune and inflammatory responses, and carcinogenesis (12,13). This pathway can reduce the occurrence of cell death by inducing the expression of anti-apoptotic protein BCL-2 and inhibiting the activation of pro-apoptotic protein BAX (12,14). Previous literature has supported the MAPK signaling pathway as a molecular target for anti-inflammatory therapy can regulate various cytokines such as TNF-α, IL-1β, IL-12 and IL-18, and the activation of p38 MAPK plays a key role in the occurrence of inflammatory reactions (15). Thus, the relationship between the MAPK/NF-κB signaling pathway and the inflammatory process is worth studying.
Isosteviol (ISV) is a derivative of steviol glycoside, which is produced by the hydrolysis of steviol glycoside through acid catalysis. Its therapeutic effect may have several mechanisms, such as antioxidation, reduction of inflammatory reaction, anti-apoptosis mechanism, and neuroprotective effect in vivo and in vitro (16-18). Some studies have shown that ISV reduced the release of pro-inflammatory cytokines and played an anti-inflammatory role by reducing the protein level of MAPK/NF-κB, which has a beneficial effect on myocardial, brain and kidney damage (19-21). The latest research on ISV reveals its involvement in multiple cellular signaling pathways, particularly in the pathogenesis of colitis. The dysregulated immune response, characterized by macrophage polarization and an imbalance of Th1/Th2/Th17/Tre cells, contributes to abnormal metabolism. In dextran sodium sulfate (DSS)-induced mice, cytokine expression levels were significantly altered compared to healthy controls, resulting in disrupted intestinal barrier integrity. Treatment with ISV demonstrated notable improvements in dysregulated metabolic profiles, primarily affecting glycerophospholipid, riboflavin, porphyrin and chlorophyll, as well as phenylalanine metabolism. Furthermore, ISV effectively enhanced the immune response and restored intestinal barrier regulation by reconstituting gut barrier integrity in mice with DSS-induced inflammatory bowel diseases (IBDs) (16), ISV exerted neuroprotection through phenotypic modulation of microglia/macrophage polarization. ISV disrupted the GAS5/miR-146a-5p axis to suppress Notch1 expression both in vivo and in vitro following stroke. Consequently, the production of pro-inflammatory cytokines was attenuated, leading to a shift towards M2 phenotype in microglia/macrophages (22).
However, there is a lack of literature to point out the therapeutic effect and potential mechanism of ISV on liver I/R injury in mice. Therefore, the main purpose of our study is to determine whether ISV has a protective effect on liver I/R injury, and assume that by inhibiting the activation of NF-κB/MAPK signaling pathway to reduce inflammatory responses and apoptosis. We present this article in accordance with the ARRIVE reporting checklist (available at https://tgh.amegroups.com/article/view/10.21037/tgh-23-66/rc).
Methods
Experimental animals
Wild-type C57BL/6 male mice, weighing 25–30 g, were purchased from the animal experiment center at 8–10 weeks of age. All the mice were housed under controlled in specific pathogen-free grades (24±2 ℃, 40–70% relative humidity, 12 h light/12 h dark cycle). Mice were free to get food and water ad libitum. A protocol was prepared before the study without registration. All animal experiments were approved by Clinical Center Laboratory Animal Welfare & Ethics Committee of Shanghai General Hospital, Shanghai Jiao Tong University (Ethical batch No. 2020AWS0032). All applicable international, national, and/or institutional guidelines for the care and use of animals were followed.
ISV reagents administration
ISV was produced by the acidic hydrolysis of stevioside (purchased from Qiushi Biotechnology Co., Ltd, Nanjing, China). Some previous studies showed that 5 mg/kg ISV had only a marginal effect on high-fat emulsion-induced hepatic aspartate aminotransferase (AST) and alanine aminotransferase (ALT) increase (23). Qiao et al. provided evidence that ISV (20 mg/kg) can reduce inflammation after burn injury by promoting an increase in the M2-classically activated (M1) macrophage ratio (24). To further examine the protective effect of ISV on hepatic I/R injury, we used the increased dosage of ISV in the present study. according to procedures previously described in the literature, the treatment group was given an intraperitoneal injection of ISV 20 mg/kg.
Mouse model of hepatic I/R
Mice do not need to fast or drink before surgery to reduce suffering and mortality and improve the success rate of the hepatic I/R model. After the mice were anesthetized with sevoflurane, the epidermis was cut along the midline of the abdomen, and then the peritoneum was cut to expose the liver, used a hemostatic clip to clamp the blood vessels supplying the left/middle liver lobe to cut off its blood supplying. After clamping for 1 h, take off the hemostatic clip to restore blood flow, close the peritoneum and epidermis, and the reperfusion time is 6 h. In the sham operation group, the blood flow was not cut off after exposing the liver in the same way, and the peritoneum was closed 1 h later. 1 mL saline was added by subcutaneous injection after the operation. Blood was collected through cardiac puncture after 6 h of reperfusion. The mice were anesthetized with sevoflurane, fixed in a supine position, and the hair on the anterior chest area was cut off. After disinfecting the skin, the peritoneum was cut open, and the right hand held with a blood sampling needle, took blood from the apex of the heart. When the puncture position was correct, the blood wound automatically enter the injector. The amount of blood was collected each time is 0.5–0.6 mL. The liver was absolutely exposed after blood collection, using ophthalmic scissors to cut the surrounding connective tissue and blood vessels, and the complete mouse liver was removed. It was washed in a sterile culture dish that had been placed with physiological saline. The left liver lobe was cut off and soaked in polyformaldehyde for fixation. The right liver lobe was frozen in a refrigerator at −80 ℃ for standby.
Experimental design
Forty-two mice were completely randomly divided into three groups: (I) sham operation group (n=6), in which mice underwent sham operation and received saline; (II) the I/R group (n=18), in which mice were subjected to hepatic I/R and received saline; (III) ISV treatment group (n=18), in which mice were subjected to hepatic I/R and 20 mg/kg of ISV solution was administered intraperitoneally after releasing the hemostatic clip that is at the time of reperfusion.
Western blots (WB)
Use protease inhibitor and RIPA lysate to lyse liver tissue, take supernatant after centrifugation, and dilute with saline to obtain liver tissue protein lysate. Use the bicinchoninic acid (BCA) assay kit (P0012S, Beyotime, Shanghai, China) to determine the protein concentration. Mix BCA reagent A and BCA reagent B in a ratio of 50:1 to form BCA working solution, and thoroughly mix. Add an appropriate amount of protein sample into a 96-well plate, then add PBS to 20 µL, finally add 200 µL of BCA working solution, place at 37 ℃ for 30 min, cool to room temperature, detect absorbance at 562 wavelengths with using an enzyme marker, and finally calculate the protein concentration value based on the standard curve made. Using p65, p38, extracellular regulated protein kinase (ERK), c-JunNH2 terminal kinase (JNK), BCL-2, BAX, caspase-3, glyceraldehyde-3-phosphate dehydrogenase (GAPDH), β-actin monoclonal antibodies (Abcam, Cambridge, UK), incubated with a concentration ratio of 1:2,000, the relative amount of protein was measured with a densitometer and expressed in absorbance units.
Enzyme-linked immunosorbent assay (ELISA)
To analyze the expression of inflammatory factors in blood and liver by using mouse TNF-α (EK282/4-48, MultiSciences, Hangzhou, China), IL-1β (EK201B/3-48, MultiSciences), IL-6 (EK206/3-98, MultiSciences) and IL-10 (M1000B, R&D Systems, Minneapolis, MN, USA) ELISA kit. Blood samples were stored at a low temperature of 4 ℃, centrifuged at 3,000 r/min for 10 min, the supernatant was stored at −80 ℃, and liver samples were simultaneously stored at −80 ℃.
Quantitative real-time polymerase chain reaction (qRT-PCR)
After extracting RNA from the liver using Trizol (#9109, TAKARA), reverse transcription of RNA (1ug) to generate cDNA, and qRT-PCR using 2× S6 Universal SYBR qPCR Mix (#Q204-02, EnzyArtisan). Reverse transcribe cDNA into complementary DNA using the following primer sequence: IL-1β, 5'-GAAATGCCACCTTTTGACAGTG-3' and 5'-TGGATGCTCTCATCAGGACAG-3'; TNF-α, 5'-CAGGCGGTGCCTATGTCTC-3' and 5'-CGATCACCCCGAAGTTCAGTAG-3'; IL-6, 5'-CTGCAAGAGACTTCCATCCAG-3' and 5'-AGTGGTATAGACAGGTCTGTTGG-3'; IL-10, 5'-CTTACTGACTGGCATGAGGATCA-3' and 5'-GCAGCTCTAGGAGCATGTGG-3'; GAPDH, 5'-TGACCTCAACTACATGGTCTACA-3' and 5'-CTTCCCATTCTCGGCCTTG-3'.
Flow cytometry analysis (FCM)
The infiltration of neutrophils and macrophages was detected by flow cytometry. Fluorescence binding PE-anti-Ly-6G (12-5931-81, eBioscience, San Diego, CA, USA) and FITC-anti-CD11b (11-0118-42, eBioscicence) antibodies were used to label neutrophils, PerCP-Cy5.5-anti-F4/80 (123127, BioLegend, San Diego, CA, USA) and FITC-anti-CD11b (11-0118-42, eBioscicence) antibodies were used to label macrophages. The collected tissue samples were analyzed by flow cytometry.
Liver myeloperoxidase (MPO) activity
MPO is a unique enzyme of neutrophils, and the detection of MPO is considered to be an excellent indicator of neutrophil activity. Use MPO kit (EK2133/2-96, MultiSciences) to detect the MPO content in serum, use 1× detection buffer: serum =1:50 for dilution, and operate according to the instructions. Detect absorbance at 450 and 570 wavelengths with using an enzyme marker.
Terminal deoxynucleotidyl transferase mediated dUTP nick-end labeling (TUNEL)
TUNEL was used to detect apoptosis. Use this technique to stain paraffin embedded liver tissue sections. Ten fields of view were assessed per section. The apoptotic index (TUNEL positive cell number/total liver cell number ×100%) was calculated for each mouse.
Annexin V probe
Annexin V is a sensitive indicator to detect early apoptosis of cells. Annexin V-FITC/PI apoptosis detection kit (CA1020-50T, Solarbio) was used. Part of the liver tissue was made into the cell suspension, and then 1× Binding Buffer buffer was used to make the suspension the that cell density was 1×106. Take 100 µL cell suspension, and add 5–15 µg purified recombinant Annexin V. Gently mix well and react at room temperature for 15 min, then add 5 µL Annexin V-FITC and 5 µL PI, gently mix well and place in a dark place at room temperature for 15 min. Add 400 µL of 1× Binding Buffer buffer to each test tube. The results were measured within 1 h using a flow cytometry.
Histopathology
The liver tissue was taken from the mouse model of hepatic I/R injury, fixed with 4% paraformaldehyde, embedded in paraffin and made into tissue sections, and stained with hematoxylin-eosin (HE). Histopathological analysis was carried out with a microscope to observe the damage of liver tissue.
Hepatocellular function
Serum AST and ALT levels are indicators of liver injury. Use AST (C009-2-1, NJJCBIO) and ALT (C010-2-1, NJJCBIO) kits to evaluate liver function. Take out serum samples from −80 ℃, and set a control hole for each group during operation. Add 20 µL of pre warmed matrix solution to the measurement hole and the control hole, and add 5 µL of serum samples to the measurement hole, do not add the control hole, and react at 37 ℃ for 30 min. Take out microporous plate and add 20 µL of the 4-dinitrophenylhydrazine solution to the hole that were used. Then add 5 µL of sample to the control hole, and react at 37 ℃ for 20 min. After taking out, add 200 µL of prepared 0.4 mol/L sodium hydroxide solution to these holes, After gently shaking and mixing, react at room temperature for 15 min, set the wavelength at 510 nm, and measure the OD value of each hole with an enzyme marker.
Statistical analysis
All experimental data are expressed as mean ± standard deviation. One-way ANOVA test was used for statistical significance, and Tukey test was used to compare the groups. The probability of P value <0.05 was considered statistically significant. Graphics and data are analyzed using GraphPad Prism software.
Results
ISV improves I/R-induced hepatocyte injury
We first investigated the effect of ISV on liver. ISV was injected into the abdominal cavity during reperfusion. The HE staining of liver samples showed that the liver tissue was severely congested, necrotic and inflammatory cells were extensively infiltrated after 6 h of reperfusion, while the area of liver necrosis and liver inflammation was significantly reduced after treatment with ISV (Figure 1A,1B). ALT and AST are important indicators to evaluate liver function, and serum ALT and AST levels reflect the severity of liver injury. Compared with the sham operated group, serum AST and ALT were significantly increased after 6 h of reperfusion, and significantly decreased after the treatment with ISV (Figure 1C). These findings confirmed that ISV can reduce liver I/R-induced injury.
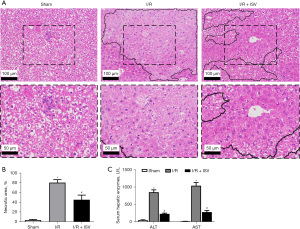
ISV can significantly attenuate mice liver tissue inflammatory response during liver I/R
We used FCM and MPO assay to detect the change of neutrophil infiltration into the liver. As shown neutrophils in the liver increased significantly after I/R, while the aggregation of neutrophils in the liver decreased significantly after treatment with ISV (Figure 2A,2B). MPO was used to accurately detect the infiltration of liver neutrophils. The activity of MPO in the liver was significantly increased after 6 h of reperfusion, and was significantly inhibited after treatment with ISV (Figure 2C). At the same time, we used FCM to detect the content of macrophages in the liver. The aggregation of macrophages was also significantly reduced after treatment with ISV (Figure 2D,2E). We detected TNF-α, IL-1β, IL-6, IL-10 and other related inflammatory factors in serum and liver tissue by ELISA and qRT-PCR respectively, the results showed that TNF-α, IL-1β, IL-6 was significantly decreased after treatment with ISV, while IL-10 expression increased (Figure 2F,2G). These findings suggest that ISV can inhibit the pro-inflammatory response and promote the anti-inflammatory response in the liver I/R injury.
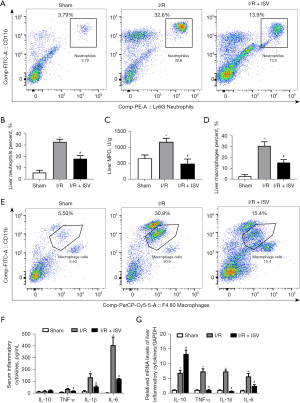
ISV suppresses mouse liver tissue apoptosis during liver I/R
The pathological process of I/R involves the occurrence of apoptosis, therefore, the activity and co-labeling of apoptosis-related proteins after treatment with ISV were detected. TUNEL immunofluorescence staining of liver and Annexin V probe detection showed that the number and area of cellular apoptosis after treatment with ISV were significantly less than those in the I/R group (Figure 3A-3D). WB showed that the expression of anti-apoptotic protein BCL-2 decreased after 6 h of reperfusion, but increased significantly in the treatment group with ISV. At the same time, the expression of pro-apoptotic protein BAX and cleaved-caspase-3 increased in the I/R group, but decreased significantly in the treatment group (Figure 3E,3F). These results show that ISV reduce the occurrence of apoptosis during hepatic I/R injury.
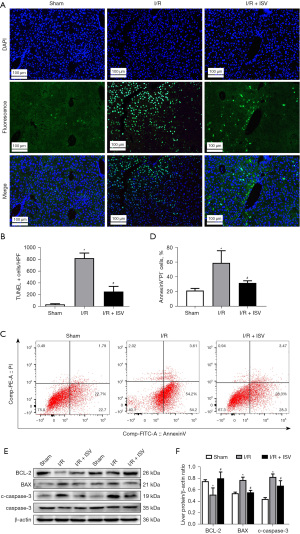
ISV plays a protective role on hepatic I/R injury by regulating the NF-κB/MAPK signaling pathway
NF-κB/MAPK signal transduction pathway is the main molecular pathway of liver disease. MAPK signal pathway includes ERK, JNK and p38, which can transcribe extracellular signals into the nucleus. In our study, we first found that the activation of p65 was significantly enhanced after 6 h of reperfusion by WB, and then we continued to study and found that the phosphorylation expression of JNK, p38 and ERK signal molecules increased after reperfusion, while the activation of these signal molecules was significantly inhibited after the treatment of ISV (Figure 4A,4B).
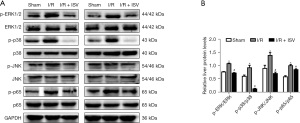
The possible mechanism of ISV treatment for hepatic I/R injury
ISV may inhibit inflammation and reduce apoptosis through the MAPK/NF-κB signaling pathway and play a protective effect against hepatic I/R injury (Figure 5).
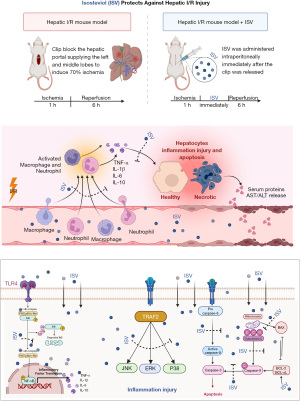
Discussion
Our study is the first to explore the positive effect of ISV on hepatic I/R injury in mice. According to the research, ISV can reduce the pro-inflammatory cytokines TNF-α, IL-1β, IL-6 and increase the release of anti-inflammatory cytokines IL-10 to reduce inflammation, simultaneously reduce the infiltration of neutrophils and the activation of macrophages. Suppressing the expression of protein related to NF-κB/MAPK signaling pathway and inhibiting hepatocyte apoptosis, thus playing an active role in the treatment process of hepatic I/R injury.
According to previous literature reports, ISV has positive anti-inflammatory and anti-apoptotic effects in the brain, intestine and heart, and has certain effects on the immune system in the body (18,25). Therefore, we tested whether it can improve liver function by using ISV in the treatment process of hepatic I/R injury. The enzyme ALT/AST in serum is a sensitive indicator of liver injury, which is significantly increased after 6 h of reperfusion, confirming that the liver injury is serious. However, the study found that the level of serum ALT and AST decreased after treatment with ISV, which confirmed that ISV had a protective effect on liver.
KCs are macrophages in the liver, and their polarization and apoptosis have been recognized as important subjects of hepatic I/R injury (1). KCs extensively gathered at the injury site during I/R and produced a large number of oxygen free radicals, which induced oxidative stress and caused liver injury (6). Activated KCs cells significantly increased the release of pro-inflammatory cytokines, including TNF-α, IL-1β and IL-6, and also promoted the recruitment and adhesion of neutrophils to the hepatic sinuses, especially TNF-α played an important role in this process (26,27). In the past decades, liver endothelial injury as the initial factor leading to hepatic I/R injury that has been widely recognized by researchers. Extensive vascular injury during reperfusion partially eliminates the liver sinus endothelial cells (LSEC) barrier, so neutrophils can enter hepatocytes directly, and the aggregation of neutrophils will eventually lead to tissue damage (28-30). During the research process, we found that macrophages and neutrophils gathered in large numbers at the liver injured site after 6 h of reperfusion, which would lead to cell inflammation and death, while the release of TNF-α, IL-1β and IL-6 was decreased, the production of anti-inflammatory IL-10 was increased in the treatment, indicating that ISV had obvious anti-inflammatory effect. Meanwhile we tested the expression of MPO in serum and found that MPO decreased significantly after ISV treatment. Reducing the activity of MPO and thereby reducing the incidence of inflammation was another important effect of ISV.
The I/R pathological process includes the occurrence of apoptosis. The reduction of neutrophils release will also reduce the release of cytokine, thus reducing cell death (27). Studies have confirmed that TNF-α promotes the occurrence of both pro-apoptotic and anti-apoptotic signals (10,31). In previous studies we had found that TNF-α elevated in hepatic I/R injury. Apoptosis is an irreversible process, and the cleavage of caspase-3 is considered to be the final and irreversible step of apoptotic cell death (10,32,33). Thus, TNF-α and caspase-3 play a key role in cell apoptosis. During the research process, we found that ISV has an obvious anti-apoptotic effect. The results showed that ISV increased the expression of the anti-apoptotic protein BCL-2 and decreased the expression of the pro-apoptotic protein cleaved-caspase-3 and BAX.
Then we investigated the possible mechanism of the protective effect of ISV on hepatic I/R injury. During reperfusion, the activation of NF-κB was increasing significantly, while NF-κB also induces the production of pro-inflammatory factor IL-1β, TNF-α (34,35). In this study, we found that treatment with ISV cound significantly inhibit the activation of NF-κB to reduce liver injury. Some studies have also confirmed that MAPK molecular signaling pathway plays a crucial role in the initiation progression and migration of liver tumors (36,37). MAPK promotes cytokines expression, such as TNF-α, IL-1β and IL-6, meanwhile activating downstream transcription factor NF-κB (34). MAPK signaling pathway has at least three different response genes that transduce extracellular signals into the nucleus, including ERK, JNK and p38 (38). MAPK-related signaling pathways are involved in hepatic I/R injury. Specifically, p38, JNK, and ERK play crucial roles in regulating liver I/R injury through various pathways such as the death receptor pathway, mitochondrial apoptosis pathway, endoplasmic reticulum reactive apoptosis pathway, and oxidative stress pathway. These signaling pathways encompass important molecules including cytochrome C, Reactive oxygen species (ROS), nitric oxide synthase, endoplasmic reticulum, TNF-α, inositol-requiring enzyme 1, secretory leukocyte protease inhibitor, superoxide dismutase, vascular cell adhesion molecule (39,40). Our results showed that the activation of p38, JNK and ERK increased in the hepatic I/R injury, and significantly inhibited the phosphorylation of p38, JNK and ERK after 6 h of treatment with ISV. The above shows that both NF-κB and MAPK signaling pathways involved in the protective effect of ISV on hepatic I/R injury. ISV reduced neutrophils flowing into the liver by inhibiting the phosphorylation of p38, JNK and ERK signal transduction, and by inhibiting the activation of NF-κB and down-regulating the expression level of pro-inflammatory cytokines to effect hepatocyte apoptosis. The above shows that both NF-κB and MAPK signaling pathways involve in the protective effect of ISV on hepatic I/R injury.
However, our research needs to be improved. The pathogenesis of I/R is diverse and complex, the relationship between ISV and MAPK/NF-κB signaling pathways is not clear, and the specific molecular mechanism needs further research.
Conclusions
ISV inhibited the activation of molecules related NF-κB and MAPK signaling pathway, inhibited the releasing of related inflammatory factors, reduced liver cellular apoptosis, and developed a good protective effect on the experimental mouse model of hepatic I/R injury.
Acknowledgments
Funding: This study was funded by
Footnote
Reporting Checklist: The authors have completed the ARRIVE reporting checklist. Available at https://tgh.amegroups.com/article/view/10.21037/tgh-23-66/rc
Data Sharing Statement: Available at https://tgh.amegroups.com/article/view/10.21037/tgh-23-66/dss
Peer Review File: Available at https://tgh.amegroups.com/article/view/10.21037/tgh-23-66/prf
Conflicts of Interest: All authors have completed the ICMJE uniform disclosure form (available at https://tgh.amegroups.com/article/view/10.21037/tgh-23-66/coif). The authors have no conflicts of interest to declare.
Ethical Statement: The authors are accountable for all aspects of the work in ensuring that questions related to the accuracy or integrity of any part of the work are appropriately investigated and resolved. All animal experiments were approved by Clinical Center Laboratory Animal Welfare & Ethics Committee of Shanghai General Hospital, Shanghai Jiao Tong University (Ethical batch No. 2020AWS0032). All applicable international, national, and/or institutional guidelines for the care and use of animals were followed.
Open Access Statement: This is an Open Access article distributed in accordance with the Creative Commons Attribution-NonCommercial-NoDerivs 4.0 International License (CC BY-NC-ND 4.0), which permits the non-commercial replication and distribution of the article with the strict proviso that no changes or edits are made and the original work is properly cited (including links to both the formal publication through the relevant DOI and the license). See: https://creativecommons.org/licenses/by-nc-nd/4.0/.
References
- Ye L, He S, Mao X, et al. Effect of Hepatic Macrophage Polarization and Apoptosis on Liver Ischemia and Reperfusion Injury During Liver Transplantation. Front Immunol 2020;11:1193. [Crossref] [PubMed]
- Guo J, Song Z, Yu J, et al. Hepatocyte-specific TMEM16A deficiency alleviates hepatic ischemia/reperfusion injury via suppressing GPX4-mediated ferroptosis. Cell Death Dis 2022;13:1072. [Crossref] [PubMed]
- G Bardallo R. Nrf2 and oxidative stress in liver ischemia/reperfusion injury. FEBS J 2022;289:5463-79. [Crossref] [PubMed]
- Michalinos A, Tsaroucha AK, Lambropoulou M, et al. Glycoprotein non-metastatic melanoma B expression after hepatic ischemia reperfusion and the effect of silibinin. Transl Gastroenterol Hepatol 2020;5:7. [Crossref] [PubMed]
- Kan C, Ungelenk L, Lupp A, et al. Ischemia-Reperfusion Injury in Aged Livers-The Energy Metabolism, Inflammatory Response, and Autophagy. Transplantation 2018;102:368-77. [Crossref] [PubMed]
- Ni M, Zhang J, Sosa R, et al. T-Cell Immunoglobulin and Mucin Domain-Containing Protein-4 Is Critical for Kupffer Cell Homeostatic Function in the Activation and Resolution of Liver Ischemia Reperfusion Injury. Hepatology 2021;74:2118-32. [Crossref] [PubMed]
- Dar WA, Sullivan E, Bynon JS, et al. Ischaemia reperfusion injury in liver transplantation: Cellular and molecular mechanisms. Liver Int 2019;39:788-801. [Crossref] [PubMed]
- Wang L, Feng ZJ, Ma X, et al. Mitochondrial quality control in hepatic ischemia-reperfusion injury. Heliyon 2023;9:e17702. [Crossref] [PubMed]
- Wu MY, Yiang GT, Liao WT, et al. Current Mechanistic Concepts in Ischemia and Reperfusion Injury. Cell Physiol Biochem 2018;46:1650-67. [Crossref] [PubMed]
- Shi JH, Sun SC. Tumor Necrosis Factor Receptor-Associated Factor Regulation of Nuclear Factor κB and Mitogen-Activated Protein Kinase Pathways. Front Immunol 2018;9:1849. [Crossref] [PubMed]
- Nennig SE, Schank JR. The Role of NFkB in Drug Addiction: Beyond Inflammation. Alcohol Alcohol 2017;52:172-9. [Crossref] [PubMed]
- Moon H, Ro SW. MAPK/ERK Signaling Pathway in Hepatocellular Carcinoma. Cancers (Basel) 2021;13:3026. [Crossref] [PubMed]
- Iroegbu JD, Ijomone OK, Femi-Akinlosotu OM, et al. ERK/MAPK signalling in the developing brain: Perturbations and consequences. Neurosci Biobehav Rev 2021;131:792-805. [Crossref] [PubMed]
- Yue J, López JM. Understanding MAPK Signaling Pathways in Apoptosis. Int J Mol Sci 2020;21:2346. [Crossref] [PubMed]
- Lucas RM, Luo L, Stow JL. ERK1/2 in immune signalling. Biochem Soc Trans 2022;50:1341-52. [Crossref] [PubMed]
- Wang S, Huang J, Liu F, et al. Isosteviol Sodium Exerts Anti-Colitic Effects on BALB/c Mice with Dextran Sodium Sulfate-Induced Colitis Through Metabolic Reprogramming and Immune Response Modulation. J Inflamm Res 2021;14:7107-30. [Crossref] [PubMed]
- Mei Y, Kuai Y, Hu H, et al. Isosteviol Sodium Attenuates High Fat/High Cholesterol-Induced Kidney Dysfunction by Inhibiting Inflammation, Oxidative Stress and Apoptosis. Biol Pharm Bull 2020;43:1172-8. [Crossref] [PubMed]
- Wang S, Tan KS, Beng H, et al. Protective effect of isosteviol sodium against LPS-induced multiple organ injury by regulating of glycerophospholipid metabolism and reducing macrophage-driven inflammation. Pharmacol Res 2021;172:105781. [Crossref] [PubMed]
- Zan J, Zhang H, Lu MY, et al. Isosteviol sodium injection improves outcomes by modulating TLRs/NF-κB-dependent inflammatory responses following experimental traumatic brain injury in rats. Neuroreport 2018;29:794-803. [Crossref] [PubMed]
- Tang SG, Liu XY, Ye JM, et al. Isosteviol ameliorates diabetic cardiomyopathy in rats by inhibiting ERK and NF-κB signaling pathways. J Endocrinol 2018;238:47-60. [Crossref] [PubMed]
- Wang S, Huang J, Tan KS, et al. Isosteviol Sodium Ameliorates Dextran Sodium Sulfate-Induced Chronic Colitis through the Regulation of Metabolic Profiling, Macrophage Polarization, and NF-κB Pathway. Oxid Med Cell Longev 2022;2022:4636618. [Crossref] [PubMed]
- Zhang H, Lu M, Zhang X, et al. Isosteviol Sodium Protects against Ischemic Stroke by Modulating Microglia/Macrophage Polarization via Disruption of GAS5/miR-146a-5p sponge. Sci Rep 2019;9:12221. [Crossref] [PubMed]
- Xu D, Xu M, Lin L, et al. The effect of isosteviol on hyperglycemia and dyslipidemia induced by lipotoxicity in rats fed with high-fat emulsion. Life Sci 2012;90:30-8. [Crossref] [PubMed]
- Qiao G, Ji W, Sun Z, et al. Isosteviol reduces the acute inflammatory response after burns by upregulating MMP9 in macrophages leading to M2 polarization. Int Immunopharmacol 2022;106:108609. [Crossref] [PubMed]
- Mohammed Abdul KS, Rayadurgam J, Faiz N, et al. Cardioprotection by isosteviol derivate JC105: A unique drug property to activate ERK1/2 only when cells are exposed to hypoxia-reoxygenation. J Cell Mol Med 2020;24:10924-34. [Crossref] [PubMed]
- Guo L, Wu X, Zhang Y, et al. Protective effects of gastrin-releasing peptide receptor antagonist RC-3095 in an animal model of hepatic ischemia/reperfusion injury. Hepatol Res 2019;49:247-55. [Crossref] [PubMed]
- Kaltenmeier C, Yazdani HO, Handu S, et al. The Role of Neutrophils as a Driver in Hepatic Ischemia-Reperfusion Injury and Cancer Growth. Front Immunol 2022;13:887565. [Crossref] [PubMed]
- Li Z, Ludwig N, Thomas K, et al. The Pathogenesis of Ischemia-Reperfusion Induced Acute Kidney Injury Depends on Renal Neutrophil Recruitment Whereas Sepsis-Induced AKI Does Not. Front Immunol 2022;13:843782. [Crossref] [PubMed]
- Kain V, Halade GV. Role of neutrophils in ischemic heart failure. Pharmacol Ther 2020;205:107424. [Crossref] [PubMed]
- Liew PX, Kubes P. The Neutrophil's Role During Health and Disease. Physiol Rev 2019;99:1223-48. [Crossref] [PubMed]
- Al-Botaty BM, Elkhoely A, K, El-Sayed E, et al. Ethyl pyruvate attenuates isoproterenol-induced myocardial infarction in rats: Insight to TNF-α-mediated apoptotic and necroptotic signaling interplay. Int Immunopharmacol 2022;103:108495. [Crossref] [PubMed]
- Schwabe RF, Luedde T. Apoptosis and necroptosis in the liver: a matter of life and death. Nat Rev Gastroenterol Hepatol 2018;15:738-52. [Crossref] [PubMed]
- Huang Q, Li F, Liu X, et al. Caspase 3-mediated stimulation of tumor cell repopulation during cancer radiotherapy. Nat Med 2011;17:860-6. [Crossref] [PubMed]
- Yu H, Lin L, Zhang Z, et al. Targeting NF-κB pathway for the therapy of diseases: mechanism and clinical study. Signal Transduct Target Ther 2020;5:209. [Crossref] [PubMed]
- Barnabei L, Laplantine E, Mbongo W, et al. NF-κB: At the Borders of Autoimmunity and Inflammation. Front Immunol 2021;12:716469. [Crossref] [PubMed]
- Guo YJ, Pan WW, Liu SB, et al. ERK/MAPK signalling pathway and tumorigenesis. Exp Ther Med 2020;19:1997-2007. [PubMed]
- Li Y, Xiong H. Correlation of LAGE3 with unfavorable prognosis and promoting tumor development in HCC via PI3K/AKT/mTOR and Ras/RAF/MAPK pathways. BMC Cancer 2022;22:298. [Crossref] [PubMed]
- Chen C, Nelson LJ, Ávila MA, et al. Mitogen-Activated Protein Kinases (MAPKs) and Cholangiocarcinoma: The Missing Link. Cells 2019;8:1172. [Crossref] [PubMed]
- Yu B, Zhang Y, Wang T, et al. MAPK Signaling Pathways in Hepatic Ischemia/Reperfusion Injury. J Inflamm Res 2023;16:1405-18. [Crossref] [PubMed]
- Ding J. Cordycepin Protects against Hepatic Ischemia/Reperfusion Injury via Inhibiting MAPK/NF-κB Pathway. Mediators Inflamm 2022;2022:5676256. [Crossref] [PubMed]
Cite this article as: Chen Y, Li R, Xu H, Guo L. Isosteviol plays a protective role on hepatic ischemia and reperfusion injury in mice through MAPK/NF-κB signaling pathway. Transl Gastroenterol Hepatol 2024;9:66.