The suppressive tumor microenvironment of AFP-positive hepatocellular carcinoma and its therapeutic implications
Hepatocellular carcinoma (HCC) is the most frequent primary liver malignancy, accounting for over 800,000 deaths worldwide (1,2). Most HCC cases arise from liver cirrhosis or metabolic dysfunction-associated steatotic liver disease (MASLD), conditions associated with severe changes in immune cell composition and signaling (3,4). The HCC tumor microenvironment (TME) is composed not only of the malignant hepatocytes, but also of resident and infiltrating leucocytes, endothelial cells, fibroblasts, and neuronal cells (5). The complex crosstalk between these cell types enables tumors to grow, to metastasize, to regulate their blood supply and to simultaneously suppress antitumor immune responses. A central mechanism in this regard is the expansion of suppressive myeloid populations, such as tumor-associated macrophages (TAM), as well as T cells with either progressive dysfunction of their cytotoxic properties or with active immunosuppressive activity (3). Interactions of immunosuppressive cell populations with each other and with malignant cells have been implicated in the response to systemic therapy and prognosis, and have resulted in increased research efforts of in-depth TME immune profiling (6,7). Immune checkpoint inhibitor (ICI) therapy has emerged as the mainstay of treatment in advanced HCC in combination with vascular endothelial growth factor (VEGF) inhibition (8), but a lack of efficient antigen presentation, immunosuppressive mechanisms and immune evasion limit response and survival rates (9). Potential second-line therapies combining ICI with other treatments, such as tyrosine kinase inhibitors, are hypothesized to have synergistic immunostimulatory effects that similarly rely on elements of antigen presentation and effector T cells (10).
Alpha-fetoprotein (AFP) is a widely employed biomarker of HCC, in a diagnostic and prognostic capacity, as well as in the longitudinal monitoring of disease (11). While the physiological function of the protein encompasses transport functions, particularly during fetal and neonatal development with large structural homology to albumin, systemically elevated AFP levels were one of the earliest documented tumor markers (12). The majority of HCCs are characterized by an overproduction of AFP and are thus considered AFP-positive HCC (APHC), while only a small fraction are AFP-negative HCCs (ANHCs) (13). A potential mechanism of AFP overexpression in HCC is DNA hypomethylation in the promoter region, a hypothesis supported by an overexpression of the DNA demethylation enzyme TET1 in these tumors (14). Elevated AFP levels are associated with aggressive clinicopathological features, more advanced HCC stages, poor differentiation, and inferior survival (14). A recent meta-analysis in HCC patients treated with ICI demonstrated that high pretreatment AFP levels were associated with shorter overall and disease-free survival, while the overall response rate was similar across AFP levels (15). At the same time, the VEGF inhibitor Ramucirumab prolonged survival as a second-line treatment in APHC (16), raising the awareness for AFP as a predictive biomarker for systemic therapy.
The recent publication by He et al. in Cell Discovery (13) extensively characterizes the TME of APHC and ANHC, shedding light on the mechanistic basis for poor prognosis in APHC and exploring the consequences for ICI therapy. In a cohort of 6 APHC and 6 ANHC, the authors undertook single-cell RNA sequencing of parenchymal and immune cells. RNA sequencing of malignant cells showed marked differences between the transcription profiles of APHC and ANHC. AFP positive tumors showed an upregulation of genes associated with antigen presentation and processing and with T-cell-tumor interactions, such as interferon (IFN) responses and human leukocyte antigen (HLA) expression. In contrast, ANHC exhibited upregulated hepatocyte-typical metabolic pathways, like bile and fatty acid metabolism. These findings could indicate a partially preserved metabolic signature of ANHC and a higher immunogenicity of APHC cells. Furthermore, a higher tumor mutational burden was found in APHC (13).
The analysis of tumor-infiltrating lymphocytes revealed an enrichment of Tregs and CD8+ exhausted T cells in APHC. In addition, the CD8+ exhausted and CD8+ cytotoxic clusters of APHC showed an enrichment of immune checkpoint-related genes that correlate with immune cell exhaustion. Accordingly, the number of checkpoint-positive [programmed cell death protein 1 (PD-1) and cytotoxic T-lymphocyte associated protein 4 (CTLA4)] T cells was higher in APHC than ANHC. CD8+ T cell-differentiation states differed between APHC and ANHC as well, with an enrichment of early-stage and exhausted CD8+ T cells in APHC, while transitional and effector states were more frequent in ANHC. He et al. additionally employed various xenograft and orthotopic chemical mouse models to recapitulate immune hallmarks of APHC and ANHC. While tumor AFP production did not impact tumor growth in immunodeficient mice, both APHC xenografts as well as adenoviral-induced AFP overexpression in chemically induced orthotopic HCC harbored a TME with an increased proportion of regulatory T cells (13).
In the myeloid compartment, APHC displayed an enrichment of SPP1+ TAMs, while dendritic cells and monocytes were more frequent in the adjacent normal tissue. Ligand-receptor analysis and in vitro experiments demonstrated that excessive stromal interleukin 6 (IL-6) and transforming growth factor beta 1 (TGF-β1) signaling induced the increased expression of SPP1 in macrophages. Those intratumoral macrophages showed an enrichment of neoangiogenesis-associated gene expression patterns and a downregulation of phagocytosis and lymphocyte recruitment. Mouse tumors artificially enriched for SPP1-deficient macrophages grew smaller in immunocompetent mice than in the presence of SPP1+ macrophages and resulted in less suppressive T cells and TAMs. As the SPP1-receptor CD44 was expressed preferentially by tumor cells of APHC, the authors investigated this axis as a therapeutic target, finding synergistic effects on tumor growth inhibition of anti-CD44/anti-Spp1 in combination with PD-1 inhibition (13). This year, the SPP1+ macrophage subset has been simultaneously identified as an essential determinant of checkpoint efficacy in HCC by another working group (17). As such, SPP1+ macrophages and fibroblasts formed a spatial tumor boundary that induced extracellular matrix remodeling and prevented lymphocyte responses. Consequently, experimental blockade of Spp1 resulted in a sensitization of HCC to ICI (17). These data corroborate the importance of SPP1+ macrophages as a key suppressive population of the HCC TME.
Overall, this study linked previous observations of tumor aggressiveness of APHC with a granular analysis of the TME and the prevailing immune responses (summarized in Figure 1). As such, APHC was associated with a higher immunogenicity, but evoked dysfunctional immune responses due to suppressive SPP1+ TAMs. Their inhibition of T cell immune responses can be potentially targeted by blocking the SPP1-CD44 axis, with synergistic effects with ICI therapy (13).
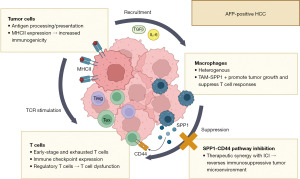
While the exact role of antigen presentation in APHC remains unclear, the increase in genes related to antigen processing and presentation as well as the increased clonal expansion of exhausted T cells fits well with the concept that differentiation of exhausted and effector T cells differs in the TME. Chronic antigen exposure and T cell receptor (TCR) signaling induce the differentiation of naïve CD8+ T cells into precursor exhausted T cells (Tpex), which activates a unique transcriptional profile driving the cells towards terminally exhausted states and preventing effector cell functions (18). Furthermore, the authors also observed an abundance of regulatory T cells in APHC, a CD4+ population with a strong immuno-suppressive role further driving cytotoxic T cell dysfunction in the TME (19).
Future studies in this direction could help linking the observed immunogenic transcription profile and increased MHCII expression of APHC with the prevailing CD8+ T cell dysfunction. Furthermore, it is imaginable that—besides TAMs—several other key populations from the TME, such as tumor cells themselves, dendritic cells (DCs) or neutrophils are also involved in dysfunctional antigen presentation in this particularly aggressive HCC subtype. For example, AFP exposure of dendritic cells has been shown to downregulate surface CD1 family proteins, which are involved in the presentation of lipid antigens to T cells (20). Immunotherapies aimed at modulating the composition of antigen-presenting cells in the TME, such as (dendritic-cell based) vaccines, might be explored to reset the TME and enable expansion of effector T cell subsets with anti-tumoral activity (9,21).
Potential research directions based on the interesting findings of He et al. could also involve the validation of their data in cohorts with other HCC etiologies. While the degree of liver steatosis is not specified for their single-cell sequencing cohort, the high number of Hepatitis B virus (HBV)-positive patients and patients with cirrhosis indicates that this cohort may be fundamentally different from American and European cohorts, where MASLD is emerging as a leading HCC tumor etiology (22,23). Recently, auto-aggressive CD8+ T cells and type 1 conventional dendritic cell overactivation have been identified as leading cancerogenous immune mechanisms of MASLD-HCC and especially as a rationale for ICI-treatment failure (24,25). Investigating the relevance of the SPP-CD44 pathway in the MASLD tumor etiology may be an interesting future step to understanding the differences between fibrosis-derived HCC and MASLD-HCC, and to optimize systemic therapy for these patients.
Acknowledgments
Funding: None.
Footnote
Provenance and Peer Review: This article was commissioned by the editorial office, Translational Gastroenterology and Hepatology. The article has undergone external peer review.
Peer Review File: Available at https://tgh.amegroups.com/article/view/10.21037/tgh-23-81/prf
Conflicts of Interest: Both authors have completed the ICMJE uniform disclosure form (available at https://tgh.amegroups.com/article/view/10.21037/tgh-23-81/coif). The authors have no conflicts of interest to declare.
Ethical Statement: The authors are accountable for all aspects of the work in ensuring that questions related to the accuracy or integrity of any part of the work are appropriately investigated and resolved.
Open Access Statement: This is an Open Access article distributed in accordance with the Creative Commons Attribution-NonCommercial-NoDerivs 4.0 International License (CC BY-NC-ND 4.0), which permits the non-commercial replication and distribution of the article with the strict proviso that no changes or edits are made and the original work is properly cited (including links to both the formal publication through the relevant DOI and the license). See: https://creativecommons.org/licenses/by-nc-nd/4.0/.
References
- Sung H, Ferlay J, Siegel RL, et al. Global Cancer Statistics 2020: GLOBOCAN Estimates of Incidence and Mortality Worldwide for 36 Cancers in 185 Countries. CA Cancer J Clin 2021;71:209-49. [Crossref] [PubMed]
- Rumgay H, Arnold M, Ferlay J, et al. Global burden of primary liver cancer in 2020 and predictions to 2040. J Hepatol 2022;77:1598-606. [Crossref] [PubMed]
- Lurje I, Hammerich L, Tacke F. Dendritic Cell and T Cell Crosstalk in Liver Fibrogenesis and Hepatocarcinogenesis: Implications for Prevention and Therapy of Liver Cancer. Int J Mol Sci 2020;21:7378. [Crossref] [PubMed]
- Lurje I, Gaisa NT, Weiskirchen R, et al. Mechanisms of organ fibrosis: Emerging concepts and implications for novel treatment strategies. Mol Aspects Med 2023;92:101191. [Crossref] [PubMed]
- Massalha H, Bahar Halpern K, Abu-Gazala S, et al. A single cell atlas of the human liver tumor microenvironment. Mol Syst Biol 2020;16:e9682. [Crossref] [PubMed]
- Mi H, Ho WJ, Yarchoan M, et al. Multi-Scale Spatial Analysis of the Tumor Microenvironment Reveals Features of Cabozantinib and Nivolumab Efficacy in Hepatocellular Carcinoma. Front Immunol 2022;13:892250. [Crossref] [PubMed]
- Ho WJ, Zhu Q, Durham J, et al. Neoadjuvant Cabozantinib and Nivolumab Converts Locally Advanced HCC into Resectable Disease with Enhanced Antitumor Immunity. Nat Cancer 2021;2:891-903. [Crossref] [PubMed]
- Awosika J, Sohal D. A narrative review of systemic treatment options for hepatocellular carcinoma: state of the art review. J Gastrointest Oncol 2022;13:426-37. [Crossref] [PubMed]
- Lurje I, Werner W, Mohr R, et al. In Situ Vaccination as a Strategy to Modulate the Immune Microenvironment of Hepatocellular Carcinoma. Front Immunol 2021;12:650486. [Crossref] [PubMed]
- Stefanini B, Ielasi L, Chen R, et al. TKIs in combination with immunotherapy for hepatocellular carcinoma. Expert Rev Anticancer Ther 2023;23:279-91. [Crossref] [PubMed]
- Johnson P, Zhou Q, Dao DY, et al. Circulating biomarkers in the diagnosis and management of hepatocellular carcinoma. Nat Rev Gastroenterol Hepatol 2022;19:670-81. [Crossref] [PubMed]
- Sauzay C, Petit A, Bourgeois AM, et al. Alpha-foetoprotein (AFP): A multi-purpose marker in hepatocellular carcinoma. Clin Chim Acta 2016;463:39-44. [Crossref] [PubMed]
- He H, Chen S, Fan Z, et al. Multi-dimensional single-cell characterization revealed suppressive immune microenvironment in AFP-positive hepatocellular carcinoma. Cell Discov 2023;9:60. [Crossref] [PubMed]
- Montal R, Andreu-Oller C, Bassaganyas L, et al. Molecular portrait of high alpha-fetoprotein in hepatocellular carcinoma: implications for biomarker-driven clinical trials. Br J Cancer 2019;121:340-3. [Crossref] [PubMed]
- Zhang L, Feng J, Kuang T, et al. Blood biomarkers predict outcomes in patients with hepatocellular carcinoma treated with immune checkpoint Inhibitors: A pooled analysis of 44 retrospective sudies. Int Immunopharmacol 2023;118:110019. [Crossref] [PubMed]
- Zhu AX, Park JO, Ryoo BY, et al. Ramucirumab versus placebo as second-line treatment in patients with advanced hepatocellular carcinoma following first-line therapy with sorafenib (REACH): a randomised, double-blind, multicentre, phase 3 trial. Lancet Oncol 2015;16:859-70. [Crossref] [PubMed]
- Liu Y, Xun Z, Ma K, et al. Identification of a tumour immune barrier in the HCC microenvironment that determines the efficacy of immunotherapy. J Hepatol 2023;78:770-82. [Crossref] [PubMed]
- Guan Q, Han M, Guo Q, et al. Strategies to reinvigorate exhausted CD8(+) T cells in tumor microenvironment. Front Immunol 2023;14:1204363. [Crossref] [PubMed]
- Granito A, Muratori L, Lalanne C, et al. Hepatocellular carcinoma in viral and autoimmune liver diseases: Role of CD4+ CD25+ Foxp3+ regulatory T cells in the immune microenvironment. World J Gastroenterol 2021;27:2994-3009. [Crossref] [PubMed]
- Li C, Song B, Santos PM, et al. Hepatocellular cancer-derived alpha fetoprotein uptake reduces CD1 molecules on monocyte-derived dendritic cells. Cell Immunol 2019;335:59-67. [Crossref] [PubMed]
- Siddiqui I, Schaeuble K, Chennupati V, et al. Intratumoral Tcf1(+)PD-1(+)CD8(+) T Cells with Stem-like Properties Promote Tumor Control in Response to Vaccination and Checkpoint Blockade Immunotherapy. Immunity 2019;50:195-211.e10. [Crossref] [PubMed]
- Paik JM, Golabi P, Biswas R, et al. Nonalcoholic Fatty Liver Disease and Alcoholic Liver Disease are Major Drivers of Liver Mortality in the United States. Hepatol Commun 2020;4:890-903. [Crossref] [PubMed]
- Lurje I, Czigany Z, Bednarsch J, et al. Treatment Strategies for Hepatocellular Carcinoma - a Multidisciplinary Approach. Int J Mol Sci 2019;20:1465. [Crossref] [PubMed]
- Pfister D, Núñez NG, Pinyol R, et al. NASH limits anti-tumour surveillance in immunotherapy-treated HCC. Nature 2021;592:450-6. [Crossref] [PubMed]
- Deczkowska A, David E, Ramadori P, et al. XCR1(+) type 1 conventional dendritic cells drive liver pathology in non-alcoholic steatohepatitis. Nat Med 2021;27:1043-54. [Crossref] [PubMed]
Cite this article as: Lurje I, Hammerich L. The suppressive tumor microenvironment of AFP-positive hepatocellular carcinoma and its therapeutic implications. Transl Gastroenterol Hepatol 2024;9:1.