Pathogenesis of non-alcoholic fatty liver disease and implications on cardiovascular outcomes in liver transplantation
The definition, magnitude, epidemiology and natural history of non-alcoholic fatty liver disease (NAFLD)
NAFLD refers to the entire spectrum of liver diseases including non-alcoholic fatty liver (NAFL) or simple steatosis, non-alcoholic steatohepatitis (NASH) with varying degrees of fibrosis including cirrhosis. It requires the presence of excessive (>5%) fat in the hepatocytes when other known causes like alcohol, defined metabolic abnormalities, endocrine diseases and medications are excluded. NASH is a subset of NAFLD accounting for about 15–20% patients with liver biopsy showing ballooning of liver cells and inflammation with varying degrees of fibrosis. NASH has a 20–30% probability of progressing to cirrhosis of the liver in 10–20 years (1-3).
With increasing obesity, about 25–30% of the population in the United States at any given time has excess fat in the liver by ultrasound—which may be an underestimate (4). NAFLD is becoming one of the most common causes for the development of cirrhosis as well as hepatocellular cancer (HCC) (5,6).
NAFLD with cirrhosis and liver failure and/or HCC has become the second most common indication for liver transplantation (LT) (7,8). NAFLD is the leading indication in those 65 years and older being listed for LT (9). Also, about 50% of “cryptogenic cirrhosis” may be due to NAFLD (10).
Cirrhosis from NAFLD is slow to develop and often remains silent until clinical signs of decompensation such as ascites, variceal bleeding, hepatic encephalopathy or HCC occurs. There are likely 2–3 million patients with cirrhosis due to NAFLD in the United States, and millions more worldwide, but the majority are unaware and undiagnosed (11).
Patients with NAFLD have multiple comorbidities that increase their risk for cardiovascular disease (CVD) including obesity, metabolic syndrome (MetS), and type 2 diabetes mellitus (T2DM). CVD is the major cause of mortality in NAFLD, even in those with early cirrhosis. Whereas, in decompensated NAFLD cirrhosis, liver related mortality predominates (12,13).
CVD in NAFLD
The mechanisms that increase CVD in patients with NAFLD are not fully clarified. Most of the patients with NAFLD have a long duration of obesity and features of insulin resistance (IR). The presence of MetS and T2DM appear to select patients with NAFL who are at increased risk for both NASH and cardiovascular complications. These patients have increased incidence of renal insufficiency which is known to further accelerate atherogenesis and CVD (14,15). In fact, NAFLD is the fastest growing indication for simultaneous liver and kidney transplantation in the United States (16).
Most studies in NAFLD-associated CVD deal with atherosclerotic disease, however, hypertensive heart disease and left ventricular diastolic dysfunction leading to heart failure (HF) increases with progression of fibrosis (17).
The cardiovascular risk of patients with NAFLD appears to be much lower in females and this appears to be due to the protective effect of estrogens. The risk of CVD in females increases after menopause, toward levels seen in males (18).
CVDs prevalent in NAFLD patients being considered for LT
The main causes of mortality in patients with non-cirrhotic NAFLD/NASH are CVD, non-liver cancers and liver related complications—in that order (19). The incidence of CVD in NAFLD has been shown to correlate with the degree of fibrosis in the liver up to cirrhosis with Child A5 stage. The liver related mortality increases in NAFLD only when patients progress to cirrhosis Child A5→A6 and beyond (12,13,19). Therefore, NAFLD-cirrhosis and NAFLD-HCC patients listed for LT are potentially at increased risk for CVD when compared to those with other etiologies.
The CVDs that are associated with NAFLD
- Related to ischemia/atherosclerosis—coronary artery disease (CAD), cerebrovascular accidents (CVA) and peripheral vascular disease (PVD). Atherosclerosis affects all large blood vessels and the severity and prevalence in NAFLD correlates with liver fibrosis progression (20-24).
- Related to left ventricular muscle remodeling leading to diastolic dysfunction and HF with preserved ejection fraction (HFpEF).
- The degree of diastolic dysfunction and impairment of exercise tolerance is directly related to the fibrosis stage in pre-cirrhotic NAFLD (25,26).
- Related to arrhythmia—QTc prolongation, atrial fibrillation (AF), ventricular arrhythmias and sudden death (26-28).
- Related to abnormal calcium deposition in the coronaries, carotids and other large arteries, mitral annular calcification and aortic sclerosis/stenosis (29-31).
Additional CVD burden related to cirrhosis—cirrhotic cardiomyopathy (CCM)
The development of cirrhosis and portal hypertension adds a whole new layer of heart problems related to increased cardiac output from peripheral and splanchnic vasodilation, changes in the pulmonary circulation and a mysterious entity called CCM. This syndrome includes a hyperdynamic circulation, a blend of systolic and diastolic dysfunction, along with prolonged ventricular repolarization, and blunted inotropic and chronotropic response to stress (32). CCM is a cardiac muscle dysfunction specific to cirrhosis and chronic liver failure that occurs de novo, but its pathophysiology and management remain unclear. It is likely a response to the vascular and neurohumoral changes occurring in cirrhosis and not an intrinsic heart disease. Once it was considered to be completely reversible with LT, but this is now being questioned (33).
CCM likely is responsible for the lion’s share of cardiovascular events that occurs during the transplant surgery and in the immediate post-operative period including HF, AF and other arrhythmias especially as we are now quite adept at managing macrovascular ischemic heart disease.
Some of the components of CCM overlap with obesity/T2DM/NAFLD related changes in the heart and this makes it even more confusing. Whether CCM is uniquely increased in NAFLD cirrhosis is not clear. Unfortunately, even with a diagnosis of CCM, we lack the ability to predict perioperative cardiac events accurately, and so the hepatologists, surgeons and anesthesiologists must stay vigilant.
Factors in causation and progression of NAFLD and their relationships to CVD
Genetics and NAFLD
Genetic mutations known to affect hepatocyte glucose and lipid metabolism have been shown to play a part in NAFLD. The mutations characterized so far include: (I) patatin-like phospholipase domain containing protein 3 (PNPLA3) variant allele rs738409—can be homozygous or heterozygous and decreases hydrolysis of stored fat; (II) transmembrane 6 superfamily member-2 (TM6SF2) mutation which decreases triglyceride incorporation into very low density lipoprotein (VLDL) for secretion (34); (III) GCKR (Glucokinase regulator) mutation rs1260326-T, associated with increased hepatic glucose uptake and causes fat accumulation along with mildly elevated VLDL and triglyceride levels (35).
These mutations appear to play a part in liver fat accumulation in “lean NAFLD” patients, but more importantly accentuates the hepatic steatosis associated with weight gain (36-39). Nevertheless, these mutations per se appear not to increase CVD (40), and in some instances may be protective, suggesting that principally, NAFLD is neither a genetic nor a primary liver disease. Acquired obesity from calorie excess and resultant NAFLD appears to be the main driver of CVD (40,41).
Role of Insulin, IR in NAFLD/MetS and CVD
The aphorism “no Insulin no fatty liver” holds true as type 1 diabetics with absolute insulin deficiency develop fatty liver only when they become overweight and and/or develop visceral adiposity associated with IR (42-44). As NAFLD evolves into NASH and fibrosis increases in the liver, IR as well as fasting Insulin levels rise concordantly.
Recent research suggests that the liver plays a major role in Insulin clearance. Sustained high carbohydrates (CHO) diet causes increased insulin production by the pancreatic β cells coupled with decreased hepatic insulin clearance and causes persistently high systemic insulin levels. This can lead to IR in the muscle and adipose tissues as an adaptation-which in turn can increase the long-term risk for NAFLD/MetS (45-47).
Tight control of blood sugar with interventions that increase blood insulin levels is associated with weight gain, and may be detrimental in those who are already metabolically unhealthy. Indeed, “intensive glucose lowering” using sulfonylureas and insulin in older, T2DM patients especially those with renal dysfunction (a population which is enriched for NAFLD/NASH) increased all-cause mortality without improving CVD outcomes and caused weight gain (48-53). Also, long-term insulin use in T2DM after cardiac stenting caused increased major adverse cardiac events (MACE) (54,55).
Adipokines and myokines in NAFLD
With IR and inflammation of white adipose tissue especially in the visceral compartment, the adipokine profile changes from the normal insulin sensitizing/anti-inflammatory to IR inducing and pro inflammatory/pro-thrombotic type and further stresses the liver (56).
Over 200 proteins and other molecules produced by the muscle called “Myokines” have been shown to influence the adipose tissue, gut microbiome and the liver. Sarcopenia widely prevalent in NAFLD can accelerate liver disease progression as well as increase CVD risks (57-59).
The gut microbiome impact on obesity, NAFLD and CVD
We know that the gut microbiome plays a role in the development and progression of NAFLD, but we are still early in our understanding. It appears that there is a complex relationship of the microbiome with the liver, adipose tissue, muscle and immune system. Altered bacterial profile (dysbiosis), a leaky gut and portal hypertension contributes to systemic inflammation. This can accelerate atherogenesis, increase cardiac muscle and electrical system dysfunction thus worsening CVD outcomes (60-63).
Genesis and evolution of NAFLD
The normal human body has no mechanism to block absorption of excess calories especially sugars and CHO. The excess calories, especially CHO goes to the liver along with increased insulin produced by the pancreas. In the liver CHO gets converted to glycogen, but this capacity is quickly exceeded. De novo lipid synthesis occurs (especially increased with fructose) and the fat generated is exported as VLDL and goes to the adipose and muscle. Eventually the adipose tissue enlarges and patients develop peripheral and/or central adiposity some of which may be genetically influenced.
NAFL or Hepatic steatosis- has been postulated as a temporary adaptive process during periods of calorie/CHO excess (64). The liver in the obese patient is faced by a triple threat of fatty acids: (I) The non-esterified fatty acids (NEFA) which accounts for about 60% of fat coming from the adipose tissue; (II) 20–30% free fatty acids (FFA) from dietary fat; (III) de novo lipogenesis (DNL) from the CHO and sugars especially fructose in the setting of very high insulin levels in the portal venous blood (65). The hepatocyte’s ability to burn adequate amount of fat and package FFA into VLDL for export to the adipose tissue and muscle is exceeded. Over time, lipotoxicity develops and this causes ballooning and death of hepatocytes with resultant inflammation (66-68). Persistence of these conditions leads to further progression of NASH through various stages of fibrosis and eventually to cirrhosis.
Liver fibrosis/cirrhosis and its impact on CVD
The inflammation in the liver from hepatocyte death causes Kupffer cell activation and also stellate cell activation which causes fibrogenesis, leading eventually to cirrhosis (69). Kupffer cells become more pro-inflammatory and react to the endotoxins and foreign proteins from the gut coming through the portal vein by releasing TNF/IL-1/IL-6/IFN-γ/IL-18 and other cytokines which contribute to systemic inflammation (70).
Prevalence of T2DM in NAFLD increases with degree of liver fibrosis, reaching over 50% in cirrhosis and may further increase CVD incidence and mortality by causing additional microvascular disease related to hyperglycemia in addition to increasing advanced glycation end products (71-73).
Increased renal dysfunction is observed in NAFLD patients especially those developing T2DM and it has been postulated that the cardiovascular outcomes are worse in this subset. However, a recent study found that the CVD risk was fully accounted for by metabolic abnormalities and renal dysfunction did not add to the risk (74,75).
Most longitudinal studies in NAFLD/NASH suggest that the cardiovascular mortality and overall mortality increase with increasing liver fibrosis which is also associated with increased IR, worsening metabolic derangements and increased systemic inflammation (76-82).
NAFLD progression increases CVD though metabolic and inflammatory components which act as proxies and can potentially explain the “honeymoon period” with decreased metabolic and CVD risks that occurs post-transplantation with a healthy new liver.
NAFLD may be the link between obesity and CVD via MetS
Most obese patients have one or more components of MetS (Table 1). These patients are categorized as metabolically unhealthy obese (MUO). While each component of MetS is a proven risk factor for CVD, having 3 or more components together constitutes the full MetS and imparts maximum CVD risk. A majority of these patients have NAFLD/NASH.
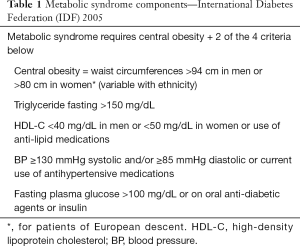
Full table
Similarly, there are patients who are “metabolically unhealthy with normal weight (MUNW)”. These patients have increased CVD similar to those who are MUO. The common factor that increases CVD in those who are metabolically unhealthy appears to be visceral and/or hepatic steatosis. There is a significant minority of patients seen in obesity clinics, who are obese, but do not have any of the components of MetS. These patients are called “metabolically healthy obese” (MHO). This phase can last for a short or long period and it appears that genetics, muscle mass and exercise likely play major roles in determining the rate of progression to MUO. Exercise in addition to burning excess calories, may by various mechanisms prevent or delay the muscle as well as adipose tissue from developing IR. Recent studies have suggested that cytokines produced by the muscle (myokines) and adipose tissue (adipokines) can also influence IR and inflammation in the liver (83). Depending on the genetic makeup of the individual, the liver may start accumulating triglycerides even at this phase as a protective adaptive mechanism to prevent toxicity of FFA within the cell.
Not so long ago MHO patients were thought to have no increase in CVD, but this has been disproven in several recent studies. NAFLD was found in 35–68% of MHO patients—which suggests NAFLD occurs much before the metabolic derangements can be detected (84,85). Most of the researchers now are of the opinion that MHO is a transitional phase with some increase in CVD risk even before it devolves into MUO (86-89). However, it is becoming increasingly clear that the presence/development of NAFLD in MHO is the one known factor that transitions them to MUO. Moreover, studies have shown that the incidence of LVH, Carotid intimal thickness and T2DM in MHO patients correlate with presence of NAFL and NASH (84,90,91).
NAFLD and MetS—the chicken or the egg?
There is confusion and controversy about whether NAFLD is the cause, manifestation or consequence of MetS, T2DM and obesity (especially visceral obesity) as these appear closely associated with each other and the interventions to treat one appear to affect all the others simultaneously (92,93).
Many leading researchers and clinicians consider NAFLD as the “hepatic manifestation of MetS” (93,94). However, longitudinal studies show that obesity—whether peripheral and/or central obesity generally precedes NAFLD which in turn precedes the development of MetS and T2DM. Also, the progression of NAFL to NASH and increasing degree of fibrosis in the liver increases the development of atherogenic dyslipidemia/MetS/T2DM (Table 2) (95).
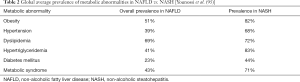
Full table
A vexing question has been whether NAFLD at various stages, increases the CVD risks over and above the traditional MetS components and this was nicely answered by a large population-based study by Allen et al., from the Mayo Clinic showed that NAFLD was an independent risk factor for metabolic comorbidities and death. The impact of NAFLD intrinsically on CVD was highest in subjects without metabolic comorbidities. The components of MetS appeared to act as proxies of NAFLD. The CVD risk of NAFLD itself waned with each additional metabolic abnormality. With full blown MetS, the CVD risks were wholly transferred to the metabolic abnormalities and NAFLD itself carried no residual risk (Figure 1) (96).
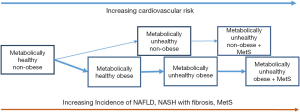
Overall, the weight of the evidence appears to suggest that obesity without NAFLD is metabolically quiescent. NAFLD is thus the connection if not the obligatory step for development of one or more metabolic abnormalities which eventually leads to MetS and simultaneously increasing the CVD risks. However, while the (diseased) liver appears to be the driver of MetS and the cardiometabolic risks in NAFLD, this does not happen in a vacuum and the individual’s genetic make-up, degree and duration of obesity (especially visceral obesity), level of muscle mass and physical activity, ongoing diet and medications can influence its character and trajectory.
Cardiovascular complications in NAFLD patients who are listed for LT
Average age of listed NAFLD cirrhosis patients is steadily increasing (9) and they have more CVD and other comorbidities than in other etiologies. Also, the rate of progression of liver disease and change of Model for End-Stage Liver Disease (MELD) scores is slower (97). Hence it is quite plausible that the waiting list mortality and dropout is higher in NAFLD cirrhosis. This was suggested in studies by Wong et al. (8) and O’Leary et al. (97). However, a more recent study by Thuluvath et al. (98) showed that the disparity of outcomes on the wait list compared to other etiologies, disappeared when clinical and demographic characteristics were factored. It is likely that due to more stringent selection criteria, a lower proportion of referred NAFLD cirrhosis patients are listed for transplantation relative to other causes.
Morbid obesity [body mass index (BMI) ≥40 kg/m2] and T2DM was shown to impact death/removal while on the wait-list in all etiologies without specific predilection to NAFLD patients (99). It was presumed that BMI ≥40 kg/m2 group would be enriched with patients who have NASH-related liver disease. However, the specific causes for the deaths on wait-list—whether they were liver related or cardiovascular was unclear.
NAFLD patients with morbid obesity and diabetes may be at increased risk for portal vein thrombosis (PVT), infections, and hepatic encephalopathy leading to increased mortality and/or drop out from the transplant waiting list. Sarcopenia is more prevalent in NAFLD cirrhosis and nutritional and physical therapy intervention may potentially decrease wait list mortality (100).
Post-LT outcomes in NAFLD
Cardiovascular outcomes post-LT in NAFLD has been a controversial subject. Despite significant increase in overall mortality and from CVD in pre-transplant NAFLD, the studies have shown good short and intermediate-term post-transplant overall survival, comparable to alcoholic and autoimmune etiologies and better than hepatitis C—for at least 5 years and some even up to about 10 years (101-104). Newer studies have tried to dissect further to see if there are differences in CVD incidence and death and whether and which pre and post-transplant factors affect it.
Early deaths and CVD post-LT
Adult patients undergoing LT appear to have increased major adverse cardiovascular and cerebrovascular events (MACCE). A recent study by Smilowitz et al. showed that perioperative MACCE occurred in 5.6% patients undergoing LT overall, compared to 2.0% in non-transplant non-cardiac surgery (105). CVD accounted for 40% of the 2.9% total early deaths and was shown to be the leading cause of 30-day mortality post-transplant in the United States by VanWagner et al. (106). Also, in the same group the 30- and 90-day hospitalizations for MACCE was increased, but over 2/3 were for AF and HF. Alcohol, NAFLD patients had increased risk of MACCE and their 1-year and long-term survival was adversely impacted (107). Baganate et al. showed 4.9% of the total transplanted died in the first 90 days and CVD was the top cause with 18.5% of these deaths. However, the 1-year mortality was similar for NAFLD vs. alcohol and autoimmune liver diseases (108).
VanWagner et al. studying 1,024 patients from a single center reported 32% MACCE with 1.7% CVD vs. 11.7% all-cause mortality in the first year post-transplantation (109). Again, AF and HF accounted for most CVD. Based on their findings, they proposed a point-based prediction model for 1-year post-transplant CVD complications using multiple pre-transplant risk factors. This has not yet been validated in other centers.
A recent study by Nagai et al., looking at the United Network for Organ Sharing (UNOS) database showed that the 1-year survival post LT for NASH cirrhosis held steady at 90.4% between 2010 and 2017, whereas during the same period, survival improved in alcoholics and hepatitis C virus (HCV) patients likely due to better medical care and access to direct acting antivirals (DAAs). Patients transplanted for NASH cirrhosis showed the highest age increase during this period. Also, while age impacted post-transplant survival in all etiologies, it was most pronounced in NASH cirrhosis and the hazard ratios for overall mortality for those above 70 years old was 2.66 when compared to those below age 50 years. Cardiac and vascular deaths were also higher in NASH cirrhosis than other etiologies (9).
What is clear from these studies is that early MACCE post-transplantation even in NAFLD is not due to CAD but from AF and HF and can increase short- and long-term morbidity and mortality. Therefore, we should more diligently assess for left ventricular diastolic dysfunction, CCM as well as for cerebrovascular disease and have a risk management plan in place.
CVD mortality beyond 1-year post-LT
Among 775 patients transplanted Cleveland Clinic, followed over 3 years, a relatively low cumulative incidence of MACCE of 10.1% was reported for the whole group, with a much higher incidence of 19.3% for NAFLD (110). CVD related deaths represented the third most common cause after infections and graft related issues. Older age at transplantation, post-transplant T2DM, Hypertension and MetS increased risk of MACCE. Overall NAFLD mortality was comparable to others
Older patients with known risk factors for CVD such as DM2, Hypertension, smoking and prior history of heart disease, likely have even higher incidence of MACCE and this has been shown by some studies (111). Other studies have shown similar or even better long-term all-cause mortality with variable CVD mortality in NAFLD (104,112).
However, looking deeper, it appears that patient age, pre-transplant T2DM and history of CAD; post-transplantation T2DM and components of MetS all increase CVD mortality as discussed below.
Pre-transplant obesity, T2DM
An older study using the patients from the UNOS database, suggested that morbid obesity decreased short- and long-term survival due to increased CVD. In a single center cohort study of 785 patients by Conzen et al., post-transplant patient and graft survival were similar across BMI categories except in those with BMI >40 kg/m2, who diverged after 3 years and had worse survival at 5 and 7 years (113). Singhal et al. showed that while morbidly obese patients have increased resource utilization post-transplantation, their short-term survival was no different (114). Barone et al. in a large meta-analysis showed that while cardiovascular complications post-transplantation was increased in patients with BMI >30 kg/m2, only the BMI >40 kg/m2 group showed consistently higher mortality at most time points after transplantation (115). The same group felt that most studies on pre-transplant obesity did not account for ascites, visceral obesity and sarcopenia and this likely caused heterogeneity in the results (116).
Unlike the above studies, pre-transplant obesity after correction for ascites did not appear to impact post-transplant survival or CVD risk as detailed by Leonard et al. looking at the NIDDK liver transplant database (117). This could also have been due to extra care taken in selecting morbidly obese patients for transplantation (118). Younossi et al. showed that pre-transplant obesity per se did not affect survival for up to 7 years post-transplantation, but pre and post-transplant DM2 in recipient increased both mortality and CVD risks (119).
Post-transplant obesity, MetS and T2DM
Obesity
Patients with NAFLD cirrhosis and HCC are often obese before they get to LT. Some patients with cirrhosis and decompensation lose weight and their BMI can decrease to underweight ranges. Most of these patients and many of those still obese also have decreased muscle mass or sarcopenia.
Post LT however, most of the patients regain weight, but because of their sedentary nature, they are slow to gain back skeletal muscle and lean body mass. Studies show that patients tend to lose average of 3–7 kg from pre-transplant weight at 3 months post-transplantation due to loss of ascites/fluid and decreased oral intake (120). After 3 months, weight gain post-transplantation was almost universal. Mean weight gain ranged 2–9 kg within the first year after transplantation (120-122). After the first year, weight gain continued, but at a slower pace up to year 3 and then plateaued but did not decrease till at least year 5. Post-transplant weight gain was highest among those who had LT vs. other solid organs (121).
Only one study assessed weight gain in NAFLD and compared it to other post-transplant patients. In this study, NAFLD patients tended to have higher mean BMI pre-LT (26 vs. 23.6 kg/m2), gained more weight over 5 years (mean weight 15 vs. 8 kg), and had a higher mean BMI increase of 5.6 vs. 2.6 over non-NAFLD patients (120).
Weight gain post-transplantation may be more harmful than pre-LT obesity. A Spanish single center cohort study from 1987 to 1997 noted that while weight gain and obesity were frequent problems after transplantation, there was no increase in morbidity and mortality secondary to CVD when compared to non-obese. Their definition of obesity was BMI >25 kg/m2 (123). A more recent prospective multicenter cohort study however showed that patients who developed new-onset obesity (defined as BMI ≥30 kg/m2) post-transplantation had a 3.5-fold increased risk of CVD mortality over a 5-year follow-up compared with patients who did not gain weight (124). The Swiss transplant cohort study showed that new onset obesity (BMI >30 kg/m2) occurred in 22% transplanted patients and MACCE in 28% over 5 years. Only new onset obesity and higher age were significant CVD risk factors, though survival was not affected by new onset obesity.
MetS
For several months before LT for NAFLD cirrhosis, most patients have low blood pressures and lipids. They also have weight loss and ascites, so it is not unexpected to have very low rates of the components of MetS. However, when these patients gain weight post-transplantation, the MetS incidence increases. The medications used for immunosuppression like steroids, calcineurin inhibitors (tacrolimus) and mTOR inhibitors (sirolimus) can cause varying levels of elevations in blood pressures, lipids and blood sugars contributing to MetS. Addition of mycophenolate to lower the dose of the other immunosuppressants may help (124).
MetS can be found in 40–60% within 5 years post-transplantation, which is about twice the prevalence in the general population in the Europe and America (125). Studies have associated MetS with pre-transplant obesity and T2DM; also, post-transplant weight gain, T2DM and recurrence or occurrence of NAFLD. MetS has been shown to increase CVD incidence post-transplantation, though not all studies show increased mortality (126-129). A systematic review by Thoefner et al. summarizes the literature with the similar conclusions (130).
T2DM post-transplantation
Patients with T2DM on treatment prior to LT in NAFLD and also other liver cirrhosis invariably redevelop diabetes post-transplantation. New onset diabetes after transplantation (NODAT) is also called post-transplant diabetes mellitus (PTDM) and it occurs in 15–30% over a period of 5 years. Andrade et al. followed 119 patients for average 4 years and found that pre-transplant T2DM (pre-DM) recurred in 100% post-transplantation. PTDM occurred in 27% and did not correlate with NAFLD, but was correlated with tacrolimus, obesity and male gender (131).
In a large single center study, Aravinthan et al. showed that CVD and renal dysfunction was higher post-transplant in those with preexisting T2DM but not in PTDM. PTDM occurred in 19% of liver transplanted patients, and 90% of them developed it within a year of transplant. Unlike the prior study, PTDM did correlate significantly with pre-transplant NAFLD. Both pre-DM and PTDM did not increase graft loss or mortality over those without DM, however, in this study, blood sugars were well controlled in both groups (132).
Two large American transplant database studies showed that pre-transplant NASH cirrhosis was linked to PTDM. Pre-DM increased CVD mortality. However, both decreased overall survival. Of interest, donor diabetes correlated with increased post-transplant graft loss as well as recipient mortality (119,133).
NAFLD post LT
De novo NAFLD and recurrent NAFLD were both closely tied to obesity and weight gain. Nearly 61% of those transplanted in the United States for NAFLD from 2008 to 2017 had BMI ≥30 kg/m2vs. 34% each for HCV and alcoholic liver disease (9). Almost all patients who engraft well, tend to gain weight post-transplantation and it is rare for even obese patients to lose weight. Lower resting and exercise energy expenditure post-transplantation maybe partly to blame (134,135). Recipient, but not donor adiponectin polymorphisms were associated with early recurrence of NAFLD (136). Whereas donor PNPLA3 genotype conferred increased risk for hepatic steatosis (137).
Among patients transplanted for NAFLD, 80–100% develop recurrence, whereas it is newly onset in 40–50% of those transplanted for other causes. Recurrent NAFLD develops quicker post-transplant and is associated with higher CVD risks over de novo NAFLD at least in the first 5 years post-transplantation, without increase in mortality (138). Some studies have suggested that the small percentage of NAFLD patients who develop NASH have poorer outcomes (139-143). In a cohort of patients transplanted for known or suspected NASH cirrhosis, Bhati et al. showed that over a median period of 47 months, 88% developed NAFLD among those biopsied with over 40% having NASH and 20% bridging fibrosis, with similar findings on transient elastography in those not biopsied. About 20% of the mortality over a 15-year period were attributed to CVD with 25% each dying from malignancy and infections respectively. No survival difference was seen between NAFLD and NASH patients (144). Unlike in the pre-transplant NAFLD population however, the cardiac and overall mortality were not shown to be related to degree of fibrosis in the liver.
In summary, over a period of 3–5 years most NAFLD patients who are transplanted develop the same cardiometabolic risk factors as in the pre-transplant period, but now they are several years older. Pre-transplant T2DM, MetS and heart disease increase the CVD risk post-transplantation in NAFLD. Recurrent NASH, MetS and T2DM post-transplantation portend increased CVD and poor long-term outcomes.
Therefore, there is good rationale for CVD being a major cause of non-graft related mortality long term in those transplanted for NAFLD and modification of known risk factors through diet, exercise and medications should be started early post-LT and continued long term with close monitoring.
Evaluation of CVD in NAFLD patients referred for LT
Atherosclerotic disease
Cardiovascular testing is done with the aim of reducing the high rate of MACCE in all phases—pre-, peri- and post-LT (145). However, the testing algorithms in pre-transplant patients are not standardized and vary from center to center. They mostly follow established perioperative CVD risk stratification like the Framingham score or ACC/ASCVD (American college of Cardiology/Atherosclerotic Cardiovascular Disease) score which focus mainly on Ischemic heart disease. Some studies have shown a strong correlation of Framingham scores with post-transplant cardiovascular events and patient survival especially when combined with renal function at the time of transplantation (146,147). Whereas other studies have shown that while CVD is a major cause of mortality, it cannot be predicted by pre-transplant evaluation of CAD (148,149).
There is so far no risk stratification specifically tailored to NAFLD patients and the International liver transplant consensus statement recently concluded that there was insufficient evidence to recommend a specific cardiovascular risk algorithm for NASH patients undergoing transplant evaluation (150).
Most centers do electrocardiogram (EKG), echocardiogram on all patients and add exercise or dobutamine stress echo cardiograms in the older lower risk patients. Those who are positive for ischemia on stress test undergo coronary angiogram and treatment as needed. Those with history of heart disease, stents/coronary artery bypass grafting (CABG) or are considered high risk by American Heart association guidelines undergo angiography (151).
More and more centers are adopting the policy of doing screening coronary angiograms in patients who have at least two of the following risk factors—older (>60 years), obese (BMI >35 kg/m2), diabetic, who have a significant history of smoking (>30 pack-year) and chronic renal insufficiency even in those with a normal stress echocardiogram, partly based on some published studies (152-155).
Whether aggressive screening for cardiovascular risk factors results in better waitlist and post-transplant survival/cardiovascular health in NAFLD is not completely clear at this time (Table 3).
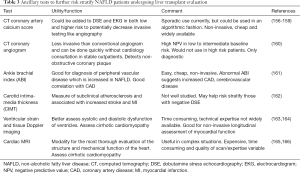
Full table
Minimizing CVD risks at various stages of NAFLD
While the hepatologist’s basic focus is on prevention of mortality from HCC and cirrhosis, we should understand that NAFLD is not an intrinsic liver disease and that when we treat it like the systemic disease it is, both liver-related and all-cause mortality can be improved.
As discussed in the pathophysiology section, the fundamental problem in NAFLD is chronic caloric excess leading to IR in the muscle, adipose tissue and the liver, which causes fatty liver and leads to MetS and chronic systemic inflammation. This basic and fundamental knowledge can be very helpful in the management of NAFLD.
This aspect is addressed in detail in another manuscript in the special edition of this journal.
Conclusions
Obesity/NAFLD epidemic continues to worsen and this will soon make NAFLD cirrhosis and HCC the most common cause for LT. NAFLD confers increased risk of cardiovascular morbidity and mortality especially when associated with MetS/T2DM. The cardiovascular and all-cause mortality appears to worsen with increasing hepatic fibrosis—likely indicating longer duration of obesity and NAFLD. Patients referred for LT with NAFLD cirrhosis/HCC are also increasing in age and need to be screened for CVD thoroughly before transplant listing. These patients need aggressive management of the risk factors and then need periodic reassessment until LT to decrease wait-list mortality and dropout.
While cardiovascular events are increased in the immediate post-operative period and early post-transplantation, the short- and intermediate-term survival is comparable to patients with other cirrhosis etiologies. Longer term however, the risk factors for CVD return or even worsen as most patients develop recurrent NAFLD/weight gain post-transplantation. Hence, to prevent CVD related complications and death, there is a need for a long-term concerted multidisciplinary effort starting around 3 months post-transplantation. It should include monitoring of nutrition indices, sarcopenia and harmful habits like smoking and alcohol intake. Dietary and exercise counseling combined with pharmacologic treatment of hypertension, T2DM, dyslipidemia is critical. Selected patients may be excellent candidates for bariatric surgery if medical/diet/exercise management fails to achieve good control of their weight and metabolic comorbidities.
Acknowledgments
None.
Footnote
Conflicts of Interest: The author has no conflicts of interest to declare.
Ethical Statement: The author is accountable for all aspects of the work in ensuring that questions related to the accuracy or integrity of any part of the work are appropriately investigated and resolved.
References
- Younossi ZM, Marchesini G, Pinto-Cortez H, et al. Epidemiology of Nonalcoholic Fatty Liver Disease and Nonalcoholic Steatohepatitis: Implications for Liver Transplantation. Transplantation 2019;103:22-7. [Crossref] [PubMed]
- Liu A, Galoosian A, Kaswala D, et al. Nonalcoholic Fatty Liver Disease: Epidemiology, Liver Transplantation Trends and Outcomes, and Risk of Recurrent Disease in the Graft. J Clin Transl Hepatol 2018;6:420-4. [Crossref] [PubMed]
- Tomic D, Kemp WW, Roberts SK. Nonalcoholic fatty liver disease: current concepts, epidemiology and management strategies. Eur J Gastroenterol Hepatol 2018;30:1103-15. [Crossref] [PubMed]
- Mishra P, Younossi ZM. Abdominal ultrasound for diagnosis of nonalcoholic fatty liver disease (NAFLD). Am J Gastroenterol 2007;102:2716-7. [Crossref] [PubMed]
- Chalasani N, Younossi Z, Lavine JE, et al. The diagnosis and management of nonalcoholic fatty liver disease: Practice guidance from the American Association for the Study of Liver Diseases. Hepatology 2018;67:328-57. [Crossref] [PubMed]
- Gitto S, Vukotic R, Vitale G, et al. Non-alcoholic steatohepatitis and liver transplantation. Dig Liver Dis 2016;48:587-91. [Crossref] [PubMed]
- Mikolasevic I, Filipec-Kanizaj T, Mijic M, et al. Nonalcoholic fatty liver disease and liver transplantation - Where do we stand? World J Gastroenterol 2018;24:1491-506. [Crossref] [PubMed]
- Wong RJ, Aguilar M, Cheung R, et al. Nonalcoholic steatohepatitis is the second leading etiology of liver disease among adults awaiting liver transplantation in the United States. Gastroenterology 2015;148:547-55. [Crossref] [PubMed]
- Nagai S, Collins K, Chau LC, et al. Increased Risk of Death in First Year After Liver Transplantation Among Patients With Nonalcoholic Steatohepatitis vs Liver Disease of Other Etiologies. Clin Gastroenterol Hepatol 2019;17:2759-2768.e5. [Crossref] [PubMed]
- Marmur J, Bergquist A, Stal P. Liver transplantation of patients with cryptogenic cirrhosis: clinical characteristics and outcome. Scand J Gastroenterol 2010;45:60-9. [Crossref] [PubMed]
- Alexander M, Loomis AK, van der Lei J, et al. Risks and clinical predictors of cirrhosis and hepatocellular carcinoma diagnoses in adults with diagnosed NAFLD: real-world study of 18 million patients in four European cohorts. BMC Med 2019;17:95. [Crossref] [PubMed]
- Sanyal AJ, Banas C, Sargeant C, et al. Similarities and differences in outcomes of cirrhosis due to nonalcoholic steatohepatitis and hepatitis C. Hepatology 2006;43:682-9. [Crossref] [PubMed]
- Ratziu V, Bonyhay L, Di Martino V, et al. Survival, liver failure, and hepatocellular carcinoma in obesity-related cryptogenic cirrhosis. Hepatology 2002;35:1485-93. [Crossref] [PubMed]
- Mikolasevic I, Racki S, Zaputovic L, et al. Nonalcoholic fatty liver disease (NAFLD) and cardiovascular risk in renal transplant recipients. Kidney Blood Press Res 2014;39:308-14. [Crossref] [PubMed]
- Papademetriou M, Athyros VG, Geladari E, et al. The Co-Existence of NASH and Chronic Kidney Disease Boosts Cardiovascular Risk: Are there any Common Therapeutic Options? Curr Vasc Pharmacol 2018;16:254-68. [Crossref] [PubMed]
- Singal AK, Hasanin M, Kaif M, et al. Nonalcoholic Steatohepatitis is the Most Rapidly Growing Indication for Simultaneous Liver Kidney Transplantation in the United States. Transplantation 2016;100:607-12. [Crossref] [PubMed]
- Chung GE, Lee JH, Lee H, et al. Nonalcoholic fatty liver disease and advanced fibrosis are associated with left ventricular diastolic dysfunction. Atherosclerosis 2018;272:137-44. [Crossref] [PubMed]
- Lonardo A, Nascimbeni F, Ballestri S, et al. Sex Differences in NAFLD: State of the Art and Identification of Research Gaps. Hepatology 2019;70:1457-69. [Crossref] [PubMed]
- Vilar-Gomez E, Calzadilla-Bertot L, Wai-Sun Wong V, et al. Fibrosis Severity as a Determinant of Cause-Specific Mortality in Patients With Advanced Nonalcoholic Fatty Liver Disease: A Multi-National Cohort Study. Gastroenterology 2018;155:443-457.e17. [Crossref] [PubMed]
- Athyros VG, Katsiki N, Karagiannis A. Nonalcoholic fatty liver disease and severity of cardiovascular disease manifestations. Angiology 2013;64:572-5. [Crossref] [PubMed]
- Chen Y, Xu M, Wang T, et al. Advanced fibrosis associates with atherosclerosis in subjects with nonalcoholic fatty liver disease. Atherosclerosis 2015;241:145-50. [Crossref] [PubMed]
- Long MT, Wang N, Larson MG, et al. Nonalcoholic fatty liver disease and vascular function: cross-sectional analysis in the Framingham heart study. Arterioscler Thromb Vasc Biol 2015;35:1284-91. [Crossref] [PubMed]
- Perelas A, Safarika V, Vlachos IS, et al. Correlation between mesenteric fat thickness and serum apolipoproteins in patients with peripheral arterial occlusive disease. Lipids Health Dis 2012;11:125. [Crossref] [PubMed]
- Hu J, Xu Y, He Z, et al. Increased risk of cerebrovascular accident related to non-alcoholic fatty liver disease: a meta-analysis. Oncotarget 2017;9:2752-60. [PubMed]
- Canada JM, Abbate A, Collen R, et al. Relation of Hepatic Fibrosis in Nonalcoholic Fatty Liver Disease to Left Ventricular Diastolic Function and Exercise Tolerance. Am J Cardiol 2019;123:466-73. [Crossref] [PubMed]
- Anstee QM, Mantovani A, Tilg H, et al. Risk of cardiomyopathy and cardiac arrhythmias in patients with nonalcoholic fatty liver disease. Nat Rev Gastroenterol Hepatol 2018;15:425-39. [Crossref] [PubMed]
- Hung CS, Tseng PH, Tu CH, et al. Nonalcoholic Fatty Liver Disease Is Associated With QT Prolongation in the General Population. J Am Heart Assoc 2015. [Crossref] [PubMed]
- Mantovani A, Rigamonti A, Bonapace S, et al. Nonalcoholic Fatty Liver Disease Is Associated With Ventricular Arrhythmias in Patients With Type 2 Diabetes Referred for Clinically Indicated 24-Hour Holter Monitoring. Diabetes Care 2016;39:1416-23. [Crossref] [PubMed]
- Mantovani A, Pernigo M, Bergamini C, et al. Heart valve calcification in patients with type 2 diabetes and nonalcoholic fatty liver disease. Metabolism 2015;64:879-87. [Crossref] [PubMed]
- Sinn DH, Kang D, Chang Y, et al. Non-alcoholic fatty liver disease and progression of coronary artery calcium score: a retrospective cohort study. Gut 2017;66:323-9. [Crossref] [PubMed]
- Remigio-Baker RA, Allison MA, Forbang NI, et al. Race/ethnic and sex disparities in the non-alcoholic fatty liver disease-abdominal aortic calcification association: The Multi-Ethnic Study of Atherosclerosis. Atherosclerosis 2017;258:89-96. [Crossref] [PubMed]
- VanWagner LB, Harinstein ME, Runo JR, et al. Multidisciplinary approach to cardiac and pulmonary vascular disease risk assessment in liver transplantation: An evaluation of the evidence and consensus recommendations. Am J Transplant 2018;18:30-42. [Crossref] [PubMed]
- Izzy M, Oh J, Watt KD. Cirrhotic Cardiomyopathy After Transplantation: Neither the Transient Nor Innocent Bystander. Hepatology 2018;68:2008-15. [Crossref] [PubMed]
- Anstee QM, Day CP. The Genetics of Nonalcoholic Fatty Liver Disease: Spotlight on PNPLA3 and TM6SF2. Semin Liver Dis 2015;35:270-90. [Crossref] [PubMed]
- Sliz E, Sebert S, Wurtz P, et al. NAFLD risk alleles in PNPLA3, TM6SF2, GCKR and LYPLAL1 show divergent metabolic effects. Hum Mol Genet 2018;27:2214-23. [Crossref] [PubMed]
- Cox AJ, Wing MR, Carr JJ, et al. Association of PNPLA3 SNP rs738409 with liver density in African Americans with type 2 diabetes mellitus. Diabetes Metab 2011;37:452-5. [Crossref] [PubMed]
- Graff M, North KE, Franceschini N, et al. PNPLA3 gene-by-visceral adipose tissue volume interaction and the pathogenesis of fatty liver disease: the NHLBI family heart study. Int J Obes (Lond) 2013;37:432-8. [Crossref] [PubMed]
- Peters HPF, Schrauwen P, Verhoef P, et al. Liver fat: a relevant target for dietary intervention? Summary of a Unilever workshop. J Nutr Sci 2017;6:e15. [Crossref] [PubMed]
- Xia MF, Lin HD, Chen LY, et al. The PNPLA3 rs738409 C>G variant interacts with changes in body weight over time to aggravate liver steatosis, but reduces the risk of incident type 2 diabetes. Diabetologia 2019;62:644-54. [Crossref] [PubMed]
- Petäjä EM, Yki-Järvinen H. Definitions of Normal Liver Fat and the Association of Insulin Sensitivity with Acquired and Genetic NAFLD-A Systematic Review. Int J Mol Sci 2016. [Crossref] [PubMed]
- Kaye SM, Maranghi M, Bogl LH, et al. Acquired liver fat is a key determinant of serum lipid alterations in healthy monozygotic twins. Obesity (Silver Spring) 2013;21:1815-22. [PubMed]
- Mantovani A, Mingolla L, Rigolon R, et al. Nonalcoholic fatty liver disease is independently associated with an increased incidence of cardiovascular disease in adult patients with type 1 diabetes. Int J Cardiol 2016;225:387-91. [Crossref] [PubMed]
- Serra-Planas E, Aguilera E, Castro L, et al. Low prevalence of non-alcoholic fatty liver disease in patients with type 1 diabetes is associated with decreased subclinical cardiovascular disease. J Diabetes 2017;9:1065-72. [Crossref] [PubMed]
- Targher G, Pichiri I, Zoppini G, et al. Increased prevalence of cardiovascular disease in Type 1 diabetic patients with non-alcoholic fatty liver disease. J Endocrinol Invest 2012;35:535-40. [PubMed]
- Bojsen-Møller KN, Lundsgaard AM, Madsbad S, et al. Hepatic Insulin Clearance in Regulation of Systemic Insulin Concentrations-Role of Carbohydrate and Energy Availability. Diabetes 2018;67:2129-36. [Crossref] [PubMed]
- Najjar SM, Perdomo G. Hepatic Insulin Clearance: Mechanism and Physiology. Physiology (Bethesda) 2019;34:198-215. [Crossref] [PubMed]
- Utzschneider KM, Kahn SE, Polidori DC. Hepatic Insulin Extraction in NAFLD Is Related to Insulin Resistance Rather Than Liver Fat Content. J Clin Endocrinol Metab 2019;104:1855-65. [Crossref] [PubMed]
- Castagno D, Baird-Gunning J, Jhund PS, et al. Intensive glycemic control has no impact on the risk of heart failure in type 2 diabetic patients: evidence from a 37,229 patient meta-analysis. Am Heart J 2011;162:938-948.e2. [Crossref] [PubMed]
- Gerstein HC, Miller ME, Byington RP, et al. Effects of intensive glucose lowering in type 2 diabetes. N Engl J Med 2008;358:2545-59. [Crossref] [PubMed]
- Papademetriou V, Lovato L, Doumas M, et al. Chronic kidney disease and intensive glycemic control increase cardiovascular risk in patients with type 2 diabetes. Kidney Int 2015;87:649-59. [Crossref] [PubMed]
- Rensing KL, Reuwer AQ, Arsenault BJ, et al. Reducing cardiovascular disease risk in patients with type 2 diabetes and concomitant macrovascular disease: can insulin be too much of a good thing? Diabetes Obes Metab 2011;13:1073-87. [Crossref] [PubMed]
- Riddle MC. Effects of intensive glucose lowering in the management of patients with type 2 diabetes mellitus in the Action to Control Cardiovascular Risk in Diabetes (ACCORD) trial. Circulation 2010;122:844-6. [Crossref] [PubMed]
- Schramm TK, Gislason GH, Kober L, et al. Diabetes patients requiring glucose-lowering therapy and nondiabetics with a prior myocardial infarction carry the same cardiovascular risk: a population study of 3.3 million people. Circulation 2008;117:1945-54. [Crossref] [PubMed]
- Kalkman DN, Woudstra P, den Heijer P, et al. One year clinical outcomes in patients with insulin-treated diabetes mellitus and non-insulin-treated diabetes mellitus compared to non-diabetics after deployment of the bio-engineered COMBO stent. Int J Cardiol 2017;226:60-4. [Crossref] [PubMed]
- Pi SH, Rhee TM, Lee JM, et al. Outcomes in Patients with Diabetes Mellitus According to Insulin Treatment After Percutaneous Coronary Intervention in the Second-Generation Drug-Eluting Stent Era. Am J Cardiol 2018;121:1505-11. [Crossref] [PubMed]
- Fujii H, Kawada N. Inflammation and fibrogenesis in steatohepatitis. J Gastroenterol 2012;47:215-25. [Crossref] [PubMed]
- Bellanti F, Romano AD, Lo Buglio A, et al. Oxidative stress is increased in sarcopenia and associated with cardiovascular disease risk in sarcopenic obesity. Maturitas 2018;109:6-12. [Crossref] [PubMed]
- Cleasby ME, Jamieson PM, Atherton PJ. Insulin resistance and sarcopenia: mechanistic links between common co-morbidities. J Endocrinol 2016;229:R67-81. [Crossref] [PubMed]
- Gusmao-Sena MH, Curvello-Silva K, Barreto-Medeiros JM, et al. Association between sarcopenic obesity and cardiovascular risk: where are we? Nutr Hosp 2016;33:592. [Crossref] [PubMed]
- Mouzaki M, Loomba R. Insights into the evolving role of the gut microbiome in nonalcoholic fatty liver disease: rationale and prospects for therapeutic intervention. Therap Adv Gastroenterol 2019;12:1756284819858470. [Crossref] [PubMed]
- Kurilshikov A, van den Munckhof ICL, Chen L, et al. Gut Microbial Associations to Plasma Metabolites Linked to Cardiovascular Phenotypes and Risk. Circ Res 2019;124:1808-20. [Crossref] [PubMed]
- Sharpton SR, Maraj B, Harding-Theobald E, Vittinghoff E, Terrault NA. Gut microbiome-targeted therapies in nonalcoholic fatty liver disease: a systematic review, meta-analysis, and meta-regression. Am J Clin Nutr. 2019. [Epub ahead of print]. [Crossref] [PubMed]
- Vallianou N, Stratigou T, Christodoulatos GS, et al. Understanding the Role of the Gut Microbiome and Microbial Metabolites in Obesity and Obesity-Associated Metabolic Disorders: Current Evidence and Perspectives. Curr Obes Rep 2019;8:317-32. [Crossref] [PubMed]
- Tsatsoulis A, Mantzaris MD, Bellou S, et al. Insulin resistance: an adaptive mechanism becomes maladaptive in the current environment - an evolutionary perspective. Metabolism 2013;62:622-33. [Crossref] [PubMed]
- Hodson L. Hepatic fatty acid synthesis and partitioning: the effect of metabolic and nutritional state. Proc Nutr Soc 2019;78:126-34. [Crossref] [PubMed]
- Donnelly KL, Smith CI, Schwarzenberg SJ, et al. Sources of fatty acids stored in liver and secreted via lipoproteins in patients with nonalcoholic fatty liver disease. J Clin Invest 2005;115:1343-51. [Crossref] [PubMed]
- Tamura S, Shimomura I. Contribution of adipose tissue and de novo lipogenesis to nonalcoholic fatty liver disease. J Clin Invest 2005;115:1139-42. [Crossref] [PubMed]
- Vedala A, Wang W, Neese RA, et al. Delayed secretory pathway contributions to VLDL-triglycerides from plasma NEFA, diet, and de novo lipogenesis in humans. J Lipid Res 2006;47:2562-74. [Crossref] [PubMed]
- Heymann F, Peusquens J, Ludwig-Portugall I, et al. Liver inflammation abrogates immunological tolerance induced by Kupffer cells. Hepatology 2015;62:279-91. [Crossref] [PubMed]
- Kazankov K, Jorgensen SMD, Thomsen KL, et al. The role of macrophages in nonalcoholic fatty liver disease and nonalcoholic steatohepatitis. Nat Rev Gastroenterol Hepatol 2019;16:145-59. [Crossref] [PubMed]
- Lonardo A, Ballestri S, Guaraldi G, et al. Fatty liver is associated with an increased risk of diabetes and cardiovascular disease - Evidence from three different disease models: NAFLD, HCV and HIV. World J Gastroenterol 2016;22:9674-93. [Crossref] [PubMed]
- Vanjiappan S, Hamide A, Ananthakrishnan R, et al. Nonalcoholic fatty liver disease in patients with type 2 diabetes mellitus and its association with cardiovascular disease. Diabetes Metab Syndr 2018;12:479-82. [Crossref] [PubMed]
- Zhou YY, Zhou XD, Wu SJ, et al. Synergistic increase in cardiovascular risk in diabetes mellitus with nonalcoholic fatty liver disease: a meta-analysis. Eur J Gastroenterol Hepatol 2018;30:631-6. [Crossref] [PubMed]
- Mantovani A, Zaza G, Byrne CD, et al. Nonalcoholic fatty liver disease increases risk of incident chronic kidney disease: A systematic review and meta-analysis. Metabolism 2018;79:64-76. [Crossref] [PubMed]
- Önnerhag K, Dreja K, Nilsson PM, et al. Increased mortality in non-alcoholic fatty liver disease with chronic kidney disease is explained by metabolic comorbidities. Clin Res Hepatol Gastroenterol 2019;43:542-50. [Crossref] [PubMed]
- Angulo P, Kleiner DE, Dam-Larsen S, et al. Liver Fibrosis, but No Other Histologic Features, Is Associated With Long-term Outcomes of Patients With Nonalcoholic Fatty Liver Disease. Gastroenterology 2015;149:389-97.e10. [Crossref] [PubMed]
- Baik M, Kim SU, Kang S, et al. Liver Fibrosis, Not Steatosis, Associates with Long-Term Outcomes in Ischaemic Stroke Patients. Cerebrovasc Dis 2019;47:32-9. [Crossref] [PubMed]
- Ekstedt M, Franzen LE, Mathiesen UL, et al. Long-term follow-up of patients with NAFLD and elevated liver enzymes. Hepatology 2006;44:865-73. [Crossref] [PubMed]
- Haflidadottir S, Jonasson JG, Norland H, et al. Long-term follow-up and liver-related death rate in patients with non-alcoholic and alcoholic related fatty liver disease. BMC Gastroenterol 2014;14:166. [Crossref] [PubMed]
- Hagström H, Nasr P, Ekstedt M, et al. Fibrosis stage but not NASH predicts mortality and time to development of severe liver disease in biopsy-proven NAFLD. J Hepatol 2017;67:1265-73. [Crossref] [PubMed]
- Stepanova M, Rafiq N, Makhlouf H, et al. Predictors of all-cause mortality and liver-related mortality in patients with non-alcoholic fatty liver disease (NAFLD). Dig Dis Sci 2013;58:3017-23. [Crossref] [PubMed]
- Younossi ZM, Stepanova M, Rafiq N, et al. Nonalcoholic steatofibrosis independently predicts mortality in nonalcoholic fatty liver disease. Hepatol Commun 2017;1:421-8. [Crossref] [PubMed]
- Oh KJ, Lee DS, Kim WK, Han BS, Lee SC, Bae KH. Metabolic Adaptation in Obesity and Type II Diabetes: Myokines, Adipokines and Hepatokines. Int J Mol Sci 2016. [Crossref] [PubMed]
- Haskins IN, Chang J, Nor Hanipah Z, et al. Patients with clinically metabolically healthy obesity are not necessarily healthy subclinically: further support for bariatric surgery in patients without metabolic disease? Surg Obes Relat Dis 2018;14:342-6. [Crossref] [PubMed]
- Huh JH, Kim KJ, Kim SU, et al. Obesity is more closely related with hepatic steatosis and fibrosis measured by transient elastography than metabolic health status. Metabolism 2017;66:23-31. [Crossref] [PubMed]
- Camhi SM, Must A, Gona PN, et al. Duration and stability of metabolically healthy obesity over 30 years. Int J Obes (Lond) 2019;43:1803-10. [Crossref] [PubMed]
- Christou KA, Christou GA, Karamoutsios A, et al. Metabolically Healthy Obesity Is Characterized by a Proinflammatory Phenotype of Circulating Monocyte Subsets. Metab Syndr Relat Disord 2019;17:259-65. [Crossref] [PubMed]
- Farello G, Antenucci A, Stagi S, et al. Metabolically healthy and metabolically unhealthy obese children both have increased carotid intima-media thickness: a case control study. BMC Cardiovasc Disord 2018;18:140. [Crossref] [PubMed]
- Feng T, Vegard M, Strand LB, et al. Metabolically Healthy Obesity and Risk for Atrial Fibrillation: The HUNT Study. Obesity (Silver Spring) 2019;27:332-8. [Crossref] [PubMed]
- Gummesson A, Stromberg U, Schmidt C, et al. Non-alcoholic fatty liver disease is a strong predictor of coronary artery calcification in metabolically healthy subjects: A cross-sectional, population-based study in middle-aged subjects. PLoS One 2018;13:e0202666. [Crossref] [PubMed]
- Hashimoto Y, Hamaguchi M, Fukuda T, et al. Fatty liver as a risk factor for progression from metabolically healthy to metabolically abnormal in non-overweight individuals. Endocrine 2017;57:89-97. [Crossref] [PubMed]
- Kim D, Touros A, Kim WR. Nonalcoholic Fatty Liver Disease and Metabolic Syndrome. Clin Liver Dis 2018;22:133-40. [Crossref] [PubMed]
- Yki-Järvinen H. Non-alcoholic fatty liver disease as a cause and a consequence of metabolic syndrome. Lancet Diabetes Endocrinol 2014;2:901-10. [Crossref] [PubMed]
- Katsiki N, Perez-Martinez P, Anagnostis P, et al. Is Nonalcoholic Fatty Liver Disease Indeed the Hepatic Manifestation of Metabolic Syndrome? Curr Vasc Pharmacol 2018;16:219-27. [Crossref] [PubMed]
- Younossi ZM, Koenig AB, Abdelatif D, et al. Global epidemiology of nonalcoholic fatty liver disease-Meta-analytic assessment of prevalence, incidence, and outcomes. Hepatology 2016;64:73-84. [Crossref] [PubMed]
- Allen AM, Therneau TM, Larson JJ, et al. Nonalcoholic fatty liver disease incidence and impact on metabolic burden and death: A 20 year-community study. Hepatology 2018;67:1726-36. [Crossref] [PubMed]
- O'Leary JG, Landaverde C, Jennings L, et al. Patients with NASH and cryptogenic cirrhosis are less likely than those with hepatitis C to receive liver transplants. Clin Gastroenterol Hepatol 2011;9:700-704.e1. [Crossref] [PubMed]
- Thuluvath PJ, Hanish S, Savva Y. Waiting List Mortality and Transplant Rates for NASH Cirrhosis When Compared With Cryptogenic, Alcoholic, or AIH Cirrhosis. Transplantation 2019;103:113-21. [Crossref] [PubMed]
- Kardashian AA, Dodge JL, Roberts J, et al. Weighing the risks: Morbid obesity and diabetes are associated with increased risk of death on the liver transplant waiting list. Liver Int 2018;38:553-63. [Crossref] [PubMed]
- Pacifico L, Perla FM, Chiesa C. Sarcopenia and nonalcoholic fatty liver disease: a causal relationship. Hepatobiliary Surg Nutr 2019;8:144-7. [Crossref] [PubMed]
- Afzali A, Berry K, Ioannou GN. Excellent posttransplant survival for patients with nonalcoholic steatohepatitis in the United States. Liver Transpl 2012;18:29-37. [Crossref] [PubMed]
- Charlton MR, Burns JM, Pedersen RA, et al. Frequency and outcomes of liver transplantation for nonalcoholic steatohepatitis in the United States. Gastroenterology 2011;141:1249-53. [Crossref] [PubMed]
- Said A. Non-alcoholic fatty liver disease and liver transplantation: outcomes and advances. World J Gastroenterol 2013;19:9146-55. [Crossref] [PubMed]
- Satapathy SK, Jiang Y, Eason JD, et al. Cardiovascular mortality among liver transplant recipients with nonalcoholic steatohepatitis in the United States-a retrospective study. Transpl Int 2017;30:1051-60. [Crossref] [PubMed]
- Smilowitz NR, Guo Y, Rao S, et al. Perioperative cardiovascular outcomes of non-cardiac solid organ transplant surgery. Eur Heart J Qual Care Clin Outcomes 2019;5:72-8. [Crossref] [PubMed]
- VanWagner LB, Lapin B, Levitsky J, et al. High early cardiovascular mortality after liver transplantation. Liver Transpl 2014;20:1306-16. [Crossref] [PubMed]
- VanWagner LB, Serper M, Kang R, et al. Factors Associated With Major Adverse Cardiovascular Events After Liver Transplantation Among a National Sample. Am J Transplant 2016;16:2684-94. [Crossref] [PubMed]
- Baganate F, Beal EW, Tumin D, et al. Early mortality after liver transplantation: Defining the course and the cause. Surgery 2018;164:694-704. [Crossref] [PubMed]
- VanWagner LB, Ning H, Whitsett M, et al. A point-based prediction model for cardiovascular risk in orthotopic liver transplantation: The CAR-OLT score. Hepatology 2017;66:1968-79. [Crossref] [PubMed]
- Albeldawi M, Aggarwal A, Madhwal S, et al. Cumulative risk of cardiovascular events after orthotopic liver transplantation. Liver Transpl 2012;18:370-5. [Crossref] [PubMed]
- Konerman MA, Fritze D, Weinberg RL, et al. Incidence of and Risk Assessment for Adverse Cardiovascular Outcomes After Liver Transplantation: A Systematic Review. Transplantation 2017;101:1645-57. [Crossref] [PubMed]
- Wang X, Li J, Riaz DR, et al. Outcomes of liver transplantation for nonalcoholic steatohepatitis: a systematic review and meta-analysis. Clin Gastroenterol Hepatol 2014;12:394-402.e1. [Crossref] [PubMed]
- Conzen KD, Vachharajani N, Collins KM, et al. Morbid obesity in liver transplant recipients adversely affects longterm graft and patient survival in a single-institution analysis. HPB (Oxford) 2015;17:251-7. [Crossref] [PubMed]
- Singhal A, Wilson GC, Wima K, et al. Impact of recipient morbid obesity on outcomes after liver transplantation. Transpl Int 2015;28:148-55. [Crossref] [PubMed]
- Barone M, Viggiani MT, Losurdo G, et al. Systematic review with meta-analysis: post-operative complications and mortality risk in liver transplant candidates with obesity. Aliment Pharmacol Ther 2017;46:236-45. [Crossref] [PubMed]
- Barone M, Viggiani MT, Avolio AW, et al. Obesity as predictor of postoperative outcomes in liver transplant candidates: Review of the literature and future perspectives. Dig Liver Dis 2017;49:957-66. [Crossref] [PubMed]
- Leonard J, Heimbach JK, Malinchoc M, et al. The impact of obesity on long-term outcomes in liver transplant recipients-results of the NIDDK liver transplant database. Am J Transplant 2008;8:667-72. [Crossref] [PubMed]
- Peck JR, Latchana N, Michaels A, et al. Diagnosis of morbid obesity may not impact healthcare utilization for orthotopic liver transplantation: A propensity matched study. World J Hepatol 2017;9:595-602. [Crossref] [PubMed]
- Younossi ZM, Stepanova M, Saab S, et al. The impact of type 2 diabetes and obesity on the long-term outcomes of more than 85 000 liver transplant recipients in the US. Aliment Pharmacol Ther 2014;40:686-94. [Crossref] [PubMed]
- Kouz J, Vincent C, Leong A, et al. Weight gain after orthotopic liver transplantation: is nonalcoholic fatty liver disease cirrhosis a risk factor for greater weight gain? Liver Transpl 2014;20:1266-74. [Crossref] [PubMed]
- Beckmann S, Nikolic N, Denhaerynck K, et al. Evolution of body weight parameters up to 3 years after solid organ transplantation: The prospective Swiss Transplant Cohort Study. Clin Transplant 2017. [Crossref] [PubMed]
- Charlton M, Rinella M, Patel D, et al. Everolimus Is Associated With Less Weight Gain Than Tacrolimus 2 Years After Liver Transplantation: Results of a Randomized Multicenter Study. Transplantation 2017;101:2873-82. [Crossref] [PubMed]
- Mazuelos F, Abril J, Zaragoza C, et al. Cardiovascular morbidity and obesity in adult liver transplant recipients. Transplant Proc 2003;35:1909-10. [Crossref] [PubMed]
- D'Avola D, Cuervas-Mons V, Marti J, et al. Cardiovascular morbidity and mortality after liver transplantation: The protective role of mycophenolate mofetil. Liver Transpl 2017;23:498-509. [Crossref] [PubMed]
- Laish I, Braun M, Mor E, et al. Metabolic syndrome in liver transplant recipients: prevalence, risk factors, and association with cardiovascular events. Liver Transpl 2011;17:15-22. [Crossref] [PubMed]
- Chang AL, Cortez AR, Bondoc A, et al. Metabolic syndrome in liver transplantation: A preoperative and postoperative concern. Surgery 2016;160:1111-7. [Crossref] [PubMed]
- García-Pajares F, Penas-Herrero I, Sanchez-Ocana R, et al. Metabolic Syndrome After Liver Transplantation: Five-Year Prevalence and Risk Factors. Transplant Proc 2016;48:3010-2. [Crossref] [PubMed]
- Madhwal S, Atreja A, Albeldawi M, et al. Is liver transplantation a risk factor for cardiovascular disease? A meta-analysis of observational studies. Liver Transpl 2012;18:1140-6. Erratum in: Liver Transpl 2013;19:113. Albeldawdi, Mazen [corrected to Albeldawi, Mazen]. [Crossref] [PubMed]
- Perito ER, Rosenthal P. Delineating definitions and risk factors for metabolic syndrome after pediatric liver transplantation. Liver Transpl 2014;20:1280. [Crossref] [PubMed]
- Thoefner LB, Rostved AA, Pommergaard HC, et al. Risk factors for metabolic syndrome after liver transplantation: A systematic review and meta-analysis. Transplant Rev (Orlando) 2018;32:69-77. [Crossref] [PubMed]
- Andrade AR, Bittencourt PL, Codes L, et al. New Onset Diabetes and Non-Alcoholic Fatty Liver Disease after Liver Transplantation. Ann Hepatol 2017;16:932-40. [Crossref] [PubMed]
- Aravinthan AD, Fateen W, Doyle AC, et al. The Impact of Preexisting and Post-transplant Diabetes Mellitus on Outcomes Following Liver Transplantation. Transplantation 2019;103:2523-30. [Crossref] [PubMed]
- Stepanova M, Henry L, Garg R, et al. Risk of de novo post-transplant type 2 diabetes in patients undergoing liver transplant for non-alcoholic steatohepatitis. BMC Gastroenterol 2015;15:175. [Crossref] [PubMed]
- Rodrigues DF, Monteze NM, Fagundes GBP, et al. Hypometabolism as a potential risk factor for overweight and obesity in liver recipients. Nutrition 2019;61:16-20. [Crossref] [PubMed]
- Singhvi A, Sadowsky HS, Cohen A, et al. Resting and Exercise Energy Metabolism After Liver Transplantation for Nonalcoholic Steatohepatitis. Transplant Direct 2017;3:e188. [Crossref] [PubMed]
- John BV, Aiken T, Garber A, et al. Recipient But Not Donor Adiponectin Polymorphisms Are Associated With Early Posttransplant Hepatic Steatosis in Patients Transplanted for Non-Nonalcoholic Fatty Liver Disease Indications. Exp Clin Transplant 2018;16:439-45. [PubMed]
- Trunečka P, Mikova I, Dlouha D, et al. Donor PNPLA3 rs738409 genotype is a risk factor for graft steatosis. A post-transplant biopsy-based study. Dig Liver Dis 2018;50:490-5. [Crossref] [PubMed]
- Vallin M, Guillaud O, Boillot O, et al. Recurrent or de novo nonalcoholic fatty liver disease after liver transplantation: natural history based on liver biopsy analysis. Liver Transpl 2014;20:1064-71. [Crossref] [PubMed]
- Newsome PN. Recurrence of nonalcoholic fatty liver disease after liver transplantation: it is common, but does it affect outcome? Liver Transpl 2010;16:420-2. [PubMed]
- Satapathy SK, Nair S, Vanatta JM. Nonalcoholic fatty liver disease following liver transplantation. Hepatol Int 2013;7:400-12. [Crossref] [PubMed]
- Merola J, Liapakis A, Mulligan DC, et al. Non-alcoholic fatty liver disease following liver transplantation: a clinical review. Clin Transplant 2015;29:728-37. [Crossref] [PubMed]
- Hejlova I, Honsova E, Sticova E, et al. Prevalence and risk factors of steatosis after liver transplantation and patient outcomes. Liver Transpl 2016;22:644-55. [Crossref] [PubMed]
- Narayanan P, Mara K, Izzy M, et al. Recurrent or De Novo Allograft Steatosis and Long-term Outcomes After Liver Transplantation. Transplantation 2019;103:e14-e21. [Crossref] [PubMed]
- Bhati C, Idowu MO, Sanyal AJ, et al. Long-term Outcomes in Patients Undergoing Liver Transplantation for Nonalcoholic Steatohepatitis-Related Cirrhosis. Transplantation 2017;101:1867-74. [Crossref] [PubMed]
- Plotkin JS, Scott VL, Pinna A, et al. Morbidity and mortality in patients with coronary artery disease undergoing orthotopic liver transplantation. Liver Transpl Surg 1996;2:426-30. [Crossref] [PubMed]
- Di Maira T, Rubin A, Puchades L, et al. Framingham score, renal dysfunction, and cardiovascular risk in liver transplant patients. Liver Transpl 2015;21:812-22. [Crossref] [PubMed]
- Romero-Cristóbal M, Mombiela T, Caballero A, et al. Clinical Utility of a Risk-Adapted Protocol for the Evaluation of Coronary Artery Disease in Liver Transplant Recipients. Liver Transpl 2019;25:1177-86. [PubMed]
- Patel SS, Lin FP, Rodriguez VA, et al. The relationship between coronary artery disease and cardiovascular events early after liver transplantation. Liver Int 2019;39:1363-71. [Crossref] [PubMed]
- Satapathy SK, Vanatta JM, Helmick RA, et al. Outcome of Liver Transplant Recipients With Revascularized Coronary Artery Disease: A Comparative Analysis With and Without Cardiovascular Risk Factors. Transplantation 2017;101:793-803. [Crossref] [PubMed]
- Tsochatzis E, Coilly A, Nadalin S, et al. International Liver Transplantation Consensus Statement on End-stage Liver Disease Due to Nonalcoholic Steatohepatitis and Liver Transplantation. Transplantation 2019;103:45-56. [Crossref] [PubMed]
- Lentine KL, Costa SP, Weir MR, et al. Cardiac disease evaluation and management among kidney and liver transplantation candidates: a scientific statement from the American Heart Association and the American College of Cardiology Foundation. J Am Coll Cardiol 2012;60:434-80. [Crossref] [PubMed]
- Grande-Trillo A, Sobrino-Marquez JM, Escobedo-Mesas E, et al. Preoperative Cardiac Assessment of Patients Undergoing Orthotopic Liver Transplantation: Experience in One Center. Transplant Proc 2015;47:2634-5. [Crossref] [PubMed]
- Maddur H, Bourdillon PD, Liangpunsakul S, et al. Role of cardiac catheterization and percutaneous coronary intervention in the preoperative assessment and management of patients before orthotopic liver transplantation. Liver Transpl 2014;20:664-72. [Crossref] [PubMed]
- Nicolau-Raducu R, Gitman M, Ganier D, et al. Adverse cardiac events after orthotopic liver transplantation: a cross-sectional study in 389 consecutive patients. Liver Transpl 2015;21:13-21. [Crossref] [PubMed]
- Snipelisky D, Levy M, Shapiro B. Utility of dobutamine stress echocardiography as part of the pre-liver transplant evaluation: an evaluation of its efficacy. Clin Cardiol 2014;37:468-72. [Crossref] [PubMed]
- Kemmer N, Case J, Chandna S, et al. The role of coronary calcium score in the risk assessment of liver transplant candidates. Transplant Proc 2014;46:230-3. [Crossref] [PubMed]
- Kong YG, Ha TY, Kang JW, et al. Incidence and Predictors of Increased Coronary Calcium Scores in Liver Transplant Recipients. Transplant Proc 2015;47:1933-8. [Crossref] [PubMed]
- Kong YG, Kang JW, Kim YK, et al. Preoperative coronary calcium score is predictive of early postoperative cardiovascular complications in liver transplant recipients. Br J Anaesth 2015;114:437-43. [Crossref] [PubMed]
- West BH, Low CG, Bista BB, et al. Significance of Coronary Artery Calcium Found on Non-Electrocardiogram-Gated Computed Tomography During Preoperative Evaluation for Liver Transplant. Am J Cardiol 2019;124:278-84. [Crossref] [PubMed]
- Poulin MF, Chan EY, Doukky R. Coronary Computed Tomographic Angiography in the Evaluation of Liver Transplant Candidates. Angiology 2015;66:803-10. [Crossref] [PubMed]
- Zou Y, Li X, Wang C, et al. Association between non-alcoholic fatty liver disease and peripheral artery disease in patients with type 2 diabetes. Intern Med J 2017;47:1147-53. [Crossref] [PubMed]
- Vidal-Perez R, Franco-Gutierrez R, Perez-Perez AJ, et al. Subclinical carotid atherosclerosis predicts all-cause mortality and cardiovascular events in obese patients with negative exercise echocardiography. World J Cardiol 2019;11:24-37. [Crossref] [PubMed]
- Møller S, Wiese S, Halgreen H, et al. Diastolic dysfunction in cirrhosis. Heart Fail Rev 2016;21:599-610. [Crossref] [PubMed]
- Rimbaş RC, Baldea SM, Guerra R, et al. New Definition Criteria of Myocardial Dysfunction in Patients with Liver Cirrhosis: A Speckle Tracking and Tissue Doppler Imaging Study. Ultrasound Med Biol 2018;44:562-74. [Crossref] [PubMed]
- Gao Y, Yang ZG, Ren Y, et al. Evaluation of myocardial fibrosis in diabetes with cardiac magnetic resonance T1-mapping: Correlation with the high-level hemoglobin A1c. Diabetes Res Clin Pract 2019;150:72-80. [Crossref] [PubMed]
- Kramer CM. Role of Cardiac MR Imaging in Cardiomyopathies. J Nucl Med 2015;56 Suppl 4:39S-45S. [Crossref] [PubMed]
Cite this article as: Maliakkal BJ. Pathogenesis of non-alcoholic fatty liver disease and implications on cardiovascular outcomes in liver transplantation. Transl Gastroenterol Hepatol 2020;5:36.