Regarding the applications of fusion-fluorescence imaging using indocyanine green in laparoscopic hepatectomy
A critical concern in performing hepatectomy for tumor resection is determining the boundaries of resection and identifying any additional lesions. Obtaining negative margins has a direct impact on disease-free survival in patients (1). The main goal is to remove neoplastic tissue while sparing sufficient normal parenchyma and preserving organ function. Deciding the location of margins in resection for liver neoplasms is based on the location of tumor. Cross-sectional imaging modalities localize the lesion and assist in pre-operative planning, but once in the operating room, the surgeon must rely on visual inspection and tactile feedback along with anatomic knowledge to localize and resect the tumor. In the setting of laparoscopic surgery, this becomes limited as bimanual palpation is no longer possible and tactile feedback becomes limited. This becomes even more so in the setting of robotic surgery as tactile feedback is absent and the surgeon must rely only on visual cues.
The use of near-infrared (NIR) fluorescence imaging technology in the operating room has greatly advanced real time intra-operative imaging and target identification. Indocyanine green (ICG), a dye traditionally used for fundoscopy and estimations of cardiac and hepatic function, is a fluorophore that emits a fluorescence signal when excited with near infrared light (750–810 nm) (2). The dye binds to plasma albumin and has been used intra-operatively to evaluate graft perfusion, aneurysm clipping, and lymphatic mapping. ICG undergoes hepatic metabolism and is excreted by hepatocytes into the biliary tract after approximately 6 h. Due to the impaired biliary excretion of hepatic malignancies compared to normal hepatocytes, ICG is preferentially retained in or around these lesions over time, allowing surgeons to visualize the fluorescent signal and identify the tumor over the liver (3).
For patients undergoing pre-operative ICG hepatic function testing several days before surgery, surface hepatic lesions can be visualized with a positive fluorescence signal. There is sufficient contrast as the ICG is retained at the lesions and ICG in the surrounding normal hepatocytes will have been excreted out in the biliary tract. The dye is versatile in that it can additionally be used as an angiographic agent when injected into portal vasculature, similar to indigo-carmine or methylene blue (4). Rather than the quick washout of the traditional dyes, the NIR signal from ICG persists throughout the surgical procedure, allowing for continued image guidance. Positive segmental staining can be obtained by selective portal vein injection while negative segmental staining can be obtained by intravenous injection during selective portal clamping. Real-time intra-operative selective segmental staining, along with contrasted-target identification, allows surgeons to more effectively resect hepatic neoplasms.
In the article titled “Applications of fusion-fluorescence imaging using indocyanine green in laparoscopic hepatectomy,” Terasawa et al. describe the use of ICG-fluorescence imaging guidance in 41 patients undergoing laparoscopic hepatectomies for a variety of malignancies (5). All patients received routine hepatic function tests with ICG 3 days prior to surgery. The retained ICG in hepatic tumors served to identify surface liver lesions during surgery. Using a laparoscopic Novadaq Pinpoint imaging system (Novadaq Technologies, Mississauga, Canada), they resected 59 lesions: 42 colorectal liver metastases, 7 hepatocellular carcinomas (HCC), 5 hemangiomas, 2 metastatic gastrointestinal stromal tumors, and 1 lymphoid cell hyperplastic nodule. As expected, none of the 6 benign tumors showed any fluorescence. Of the remaining 53 malignant lesions, only 23 (43%) were visible over the liver surface using bright light imaging whereas 45 (85%) were detected using fluorescence imaging. In addition, the fluorescence-navigation technology visualized colorectal-cancer liver metastases that appeared to have undergone complete radiographic regression on pre-operative imaging. The lesions were not detectable with bright light imaging or using contrasted intra-operative ultrasound, but when resected were positive for malignant cells on pathology. If a portal segment or sub-segment needed to be removed, ICG was given intravenously with the appropriate portal pedicle clamped. The demarcation between the non-fluorescing liver segment containing the tumor and the fluorescent hepatic parenchyma was visualized to help guide boundaries.
During these procedures, Terasawa et al. use a composite video feed created from the superimposition of pseudocolor-fluorescence information on white-light color video feed. This approach has been termed “fusion ICG-fluorescence imaging”. Rather than switching back and forth from fluorescent mode to bright field color mode, the surgeon can see bright-light surgical field, augmented with additional information from the fluorescence signal merged together in one screen. They report that using fusion ICG-fluorescence imaging continuously throughout parenchymal transection was feasible and safe for the patient. They especially commented that it has the potential to detect tumors on the liver surface. The continuous use of this enhanced surgical field could allow surgeons to more fluidly proceed with resection, while maximizing visualization without distracting shifts in the surgical field.
The integration of fluorescence-navigation technology in hepatectomies has been rapid. A large body of knowledge has been developed in its application, pioneered by surgeons in Japan. In 2008, Aoki et al. first described successfully using ICG as an angiographic agent, to outline liver segments after intraoperative selective portal vein injection (6). The following year, Gotoh et al. first used ICG fluorescence to localize and navigate resection margins for HCC lesions in patients receiving intravenous ICG as part of a routine pre-operative liver function assessment (7). Uchiyama et al. first used ICG fluorescence in localizing colorectal-cancer liver metastases and found that even lesions that were radiographically invisible after neoadjuvant chemotherapy still showed a positive fluorescent signal and were found to be positive for microscopically viable cancer cells on (8). Use of fluorescence navigation technology has been increasingly used for liver surgery in a relatively short period of time.
NIR fluorescence navigation is well-suited for minimally invasive hepatectomies, as it is already a setting where the surgeon uses a monitor with a video feed to interact with the surgical field. Fluorescence contrast enhancement assists in compensating for the limited ability to perform complete visual inspection and palpation of the liver during laparoscopy. NIR fluorescence laparoscopes were commercially available in 2011, only 4 years after introduction of the technology to open surgery. Ishizawa et al. first reported the initial experience in 2012 on the use of laparoscopic fluorescence imaging guidance in staining of hepatic segments during resection (9). Kudo et al. reported the first series of patients undergoing laparoscopic hepatectomy using a positive fluorescence signal to identify liver-surface tumors (10). Fluorescence navigation technology successfully assisted in determining the location of appropriate resection margins in minimally invasive settings.
First generation fluorescence imaging devices displayed black and white video on the screen. The positive fluorescence signal was usually displayed in white and the negative background in black. Pseudo-color application of the black and white images was also used. In open hepatectomies, the surgeon actively switches between using the on-screen fluorescence-image guidance for navigation and interacting with the open surgical field. In laparoscopic surgery, the surgeon had to switch between the fluorescence mode for navigation and the standard white light color video feed for surgery. Often, the resolution and video quality of fluorescence laparoscopes were sub-optimal compared to standard high-definition laparoscopes. This led to laparoscopic surgeons having two different laparoscopic systems on the operating field. Not only were the surgeons toggling between two different images, they were switching between two systems completely. The fluorescence signal was visualized in real-time, but navigation was used intermittently.
Newer fluorescence imaging devices have since arrived on the market. The upgraded optical quality and resolution in the color video feed, along with improved software allows a pseudo-color overlay channel. This fusion-fluorescence imaging, as detailed in Dr. Terasawa’s paper, allows the surgeon to use information from the fluorescence signal more fluidly throughout the procedure. Similar to the concept of augmented reality-image overlay using three-dimensional rendering from pre-operative imaging, the process attempts to create a surgical field with enhanced visual-feedback information to the surgeon in a limited environment (11). While this approach has significant potential, it remains to be seen whether it will become more widely adopted, or whether surgeons will continue to switch back and forth, finding instead, that the continuous feed of additional information is itself distracting.
Fusion ICG-fluorescence imaging is very promising in its integration of information for surgical navigation, but as its NIR fluorescent imaging predecessors, the imaging systems and their use of a non-specific fluorescent dye has a critical drawback that must be discussed. From a biologic standpoint, not all nodules that pass a software threshold for a fluorescence signal are cancer. While the information on ICG fluorescence patterns is accumulating, there is not one signal intensity or pattern that definitely indicates malignancy. It is not a major issue for fluorescent lesions that correlate appropriately with pre-operative imaging.
However, intraoperative fluorescence imaging often identifies additional fluorescent lesions that are not otherwise detected on pre-operative imaging or intra-operative ultrasound. When these lesions are identified and resected, not all are malignant on pathology. Case series reporting on additional lesions discovered by NIR fluorescence imaging have commented on falsely-positive fluorescent nodules, especially in the setting of cirrhosis. False-positive rates are variable and can be as high as 40% in all comers and up to 87% in cirrhotic livers (12). In Terasawa’s paper the group does not resect all fluorescent lesions unless a combination of clinical judgment, suspicion on white light inspection, and contrast-enhanced intraoperative ultrasound suggested malignancy. The non-specific nature of ICG plasma protein binding and hepatic excretion leads to this inherent weakness.
Tumor-specific fluorescent probes have been used in in vitro research for imaging cellular and sub-cellular structures for many years. Only recently, have they been adapted for in vivo fluorescence imaging. Targeting antigen-antibody binding, peptide binding, selective protease activation, pH differential, or aberrant tumor metabolism allows delivery of fluorescence signal specifically to target tumors with limited off target effects.
Our laboratory has shown in mouse models, that tumor-specific fluorescence labeling of tumors allows identification of even sub-millimeter lesions in laparoscopy (13). We have developed an orthotopic colon-cancer liver metastasis model and have shown improved outcomes after resection using fluorescence labeling (14). Colon cancer liver metastases were modeled by serially selecting for aggressive liver metastasis variants of colon cancer cells after splenic injection. These cells were then orthotopically implanted into the liver of recipient nude mice to model individual colon cancer metastatic nodules. Tumor-specific fluorescence was delivered in situ via viral vector expressing green fluorescent protein (GFP) or a fluorescently labeled antibody. Yano et al. and Kishimoto et al. used OBP-401, a conditionally replicative adenovirus with the replication cassette under the control of the human telomerase reverse transcriptase (hTERT) promoter and a GFP tag under the control of a cytomegalovirus (CMV) promoter (14,15). Three days after direct intratumoral injection of the adenovirus, a strong GFP signal was present at the tumor site and the fluorescence signal was used for fluorescence-guided surgery (FGS). Compared to standard bright light surgery (BLS), 15/16 (94%) of mice that underwent BLS had large tumor recurrences vs. FGS 3/16 (19%). Hiroshima et al. also compared FGS vs. BLS using an orthotopic colon cancer liver metastasis model, but delivered fluorescence with an intravenous injection of anti-CEA antibody conjugated to a 650 nm fluorophore (16). The antibody-fluorophore conjugate was successful at delineating the tumor over the liver with a best signal-to-noise ratio at 72 h. Additionally, the increased wavelength of the dye showed an improved fluorescence signal penetration in the presence of overlying tissue compared to GFP (Figure 1). Disease-free and overall survival was significantly longer in the FGS group compared to BLS. In these orthotopic mouse models of colon-cancer liver metastases, using tumor-specific fluorescence navigation for surgical resection decreased the volume of residual disease and increased overall and disease-free survival.
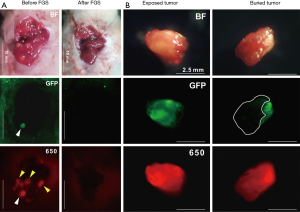
Clinical translation of these approaches was first pioneered by van Dam et al. in 2011 using folate conjugated to a fluorescein isothiocyanate (FITC) dye to target folate receptor overexpression in ovarian cancer (17). The median number of lesions identified by the fluorescein dye was higher than with bright light alone (34 vs. 7). All fluorescent lesions were shown to be malignant ovarian cancer and over expressed folate receptors. All tissue with a negative fluorescence signal was either benign or did not express folate receptors.
Other groups have also advanced into this field with development of novel fluorescent probes in clinical trials. Unkart et al. have completed a phase I trial on an activatable cell-penetrating peptides that produce a fluorescence signal upon proteolytic cleave age by matrix metalloproteases in breast cancer (NCT02391194) (18). Rosenthal et al. have published on phase I/II clinical trials of an anti-EGFR antibody (cetuximab) conjugated to an 800 nm dye to successfully image margins of head and neck cancers (NCT01987375) (19,20). Terwisscha van Scheltinga et al. used an anti-VEGF antibody (bevacizumab) and an anti-HER2 antibody (trastuzumab) conjugated to an 800 nm dye to label breast and gastric cancer in mice (21). Phase I/II clinical trials of this fluorescent anti-VEGF antibody have completed clinical trials in breast cancer (NCT02583568) and are ongoing in pancreatic cancer (NCT02743975). Gutowski et al. reported an anti-CEA antibody conjugated to a 700 nm dye that successfully image colorectal and pancreatic cancers in mice (22). This probe is currently completing phase I/II clinical trials for colorectal cancers and pancreatic cancers, but was found also to be able to label liver metastases from colorectal cancer (NCT02973672). While these novel agents are still relatively early in their development, the preliminary results show that they are promising agents, greatly improved in their fluorescence-signal delivery to their target lesions, compared to a nonspecific dye such as ICG. The number of targeted fluorescence imaging probes will greatly increase in the future.
Determination of margins in both open and minimally invasive hepatectomies has been greatly improved by the use of fluorescence imaging. The technology allows surgeons to better identify the tumor, delineate safe boundaries of resections, improve preservation of normal hepatic parenchyma, and identify additional lesions that would otherwise have been missed with only bright-light visualization. However, the technology has a critical drawback in its lack of tumor specificity. This lack of fluorescence-signal specificity is actively being addressed with novel targeted probes, several of which are progressing through phase I/II clinical trials. The field of fluorescence-guided hepatectomy, especially minimally invasive hepatectomy, is poised to greatly benefit from advancements in technology, leading surgeons to perform more oncologically-complete and safe tumor resections.
Acknowledgements
Funding: TM Lwin was funded by the NIH/NCI Award T32CA121938; M Bouvet and AntiCancer, Inc. were funded in part by National Cancer Institute; grant numbers: CA126023 and CA142669.
Footnote
Conflicts of Interest: RM Hoffman is a non-salaried associate of AntiCancer, Inc. The other authors have no conflicts of interest to declare.
References
- Pawlik TM, Scoggins CR, Zorzi D, et al. Effect of surgical margin status on survival and site of recurrence after hepatic resection for colorectal metastases. Ann Surg 2005;241:715-22, discussion 722-4. [Crossref] [PubMed]
- Jonak C, Skvara H, Kunstfeld R, et al. Intradermal indocyanine green for in vivo fluorescence laser scanning microscopy of human skin: a pilot study. PLoS One 2011;6:e23972. [Crossref] [PubMed]
- Schaafsma BE, Mieog JS, Hutteman M, et al. The clinical use of indocyanine green as a near-infrared fluorescent contrast agent for image-guided oncologic surgery. J Surg Oncol 2011;104:323-32. [Crossref] [PubMed]
- Kobayashi Y, Kawaguchi Y, Kobayashi K, et al. Portal vein territory identification using indocyanine green fluorescence imaging: Technical details and short-term outcomes. J Surg Oncol 2017. [Epub ahead of print]. [Crossref] [PubMed]
- Terasawa M, Ishizawa T, Mise Y, et al. Applications of fusion-fluorescence imaging using indocyanine green in laparoscopic hepatectomy. Surg Endosc 2017. [Epub ahead of print]. [Crossref] [PubMed]
- Aoki T, Yasuda D, Shimizu Y, et al. Image-guided liver mapping using fluorescence navigation system with indocyanine green for anatomical hepatic resection. World J Surg 2008;32:1763-7. [Crossref] [PubMed]
- Gotoh K, Yamada T, Ishikawa O, et al. A novel image-guided surgery of hepatocellular carcinoma by indocyanine green fluorescence imaging navigation. J Surg Oncol 2009;100:75-9. [Crossref] [PubMed]
- Uchiyama K, Ueno M, Ozawa S, et al. Combined use of contrast-enhanced intraoperative ultrasonography and a fluorescence navigation system for identifying hepatic metastases. World J Surg 2010;34:2953-9. [Crossref] [PubMed]
- Ishizawa T, Zuker NB, Kokudo N, et al. Positive and negative staining of hepatic segments by use of fluorescent imaging techniques during laparoscopic hepatectomy. Arch Surg 2012;147:393-4. [Crossref] [PubMed]
- Kudo H, Ishizawa T, Tani K, et al. Visualization of subcapsular hepatic malignancy by indocyanine-green fluorescence imaging during laparoscopic hepatectomy. Surg Endosc 2014;28:2504-8. [Crossref] [PubMed]
- Pessaux P, Diana M, Soler L, et al. Towards cybernetic surgery: robotic and augmented reality-assisted liver segmentectomy. Langenbecks Arch Surg 2015;400:381-5. [Crossref] [PubMed]
- Tanaka T, Takatsuki M, Hidaka M, et al. Is a fluorescence navigation system with indocyanine green effective enough to detect liver malignancies? J Hepatobiliary Pancreat Sci 2014;21:199-204. [Crossref] [PubMed]
- Tran Cao HS, Kaushal S, Metildi CA, et al. Tumor-specific fluorescence antibody imaging enables accurate staging laparoscopy in an orthotopic model of pancreatic cancer. Hepatogastroenterology 2012;59:1994-9. [PubMed]
- Yano S, Takehara K, Miwa S, et al. Improved Resection and Outcome of Colon-Cancer Liver Metastasis with Fluorescence-Guided Surgery Using In Situ GFP Labeling with a Telomerase-Dependent Adenovirus in an Orthotopic Mouse Model. PLoS One 2016;11:e0148760. [Crossref] [PubMed]
- Kishimoto H, Zhao M, Hayashi K, et al. In vivo internal tumor illumination by telomerase-dependent adenoviral GFP for precise surgical navigation. Proc Natl Acad Sci U S A 2009;106:14514-7. [Crossref] [PubMed]
- Hiroshima Y, Lwin TM, Murakami T, et al. Effective fluorescence-guided surgery of liver metastasis using a fluorescent anti-CEA antibody. J Surg Oncol 2016;114:951-8. [Crossref] [PubMed]
- van Dam GM, Themelis G, Crane LM, et al. Intraoperative tumor-specific fluorescence imaging in ovarian cancer by folate receptor-α targeting: first in-human results. Nat Med 2011;17:1315-9. [Crossref] [PubMed]
- Unkart JT, Chen SL, Wapnir IL, et al. Intraoperative Tumor Detection Using a Ratiometric Activatable Fluorescent Peptide: A First-in-Human Phase 1 Study. Ann Surg Oncol 2017. [Epub ahead of print].
- Rosenthal EL, Warram JM, de Boer E, et al. Safety and Tumor Specificity of Cetuximab-IRDye800 for Surgical Navigation in Head and Neck Cancer. Clin Cancer Res 2015;21:3658-66. [Crossref] [PubMed]
- Rosenthal EL, Moore LS, Tipirneni K, et al. Sensitivity and Specificity of Cetuximab-IRDye800CW to Identify Regional Metastatic Disease in Head and Neck Cancer. Clin Cancer Res 2017;23:4744-52. [Crossref] [PubMed]
- Terwisscha van Scheltinga AG, van Dam GM, Nagengast WB, et al. Intraoperative near-infrared fluorescence tumor imaging with vascular endothelial growth factor and human epidermal growth factor receptor 2 targeting antibodies. J Nucl Med 2011;52:1778-85. [Crossref] [PubMed]
- Gutowski M, Framery B, Boonstra MC, et al. SGM-101: An innovative near-infrared dye-antibody conjugate that targets CEA for fluorescence-guided surgery. Surg Oncol 2017;26:153-62. [Crossref] [PubMed]
Cite this article as: Lwin TM, Hoffman RM, Bouvet M. Regarding the applications of fusion-fluorescence imaging using indocyanine green in laparoscopic hepatectomy. Transl Gastroenterol Hepatol 2017;2:70.